Translate this page into:
Lamotrigine effects sensorimotor gating in WAG/Rij rats
Address for correspondence: Dr. İpek Komsuoglu Celikyurt, Pharmacology Department, Psychopharmacology Laboratory, Kocaeli University, Kocaeli, Turkey E-mail: ikomsu@hotmail.com
This is an open-access article distributed under the terms of the Creative Commons Attribution-Noncommercial-Share Alike 3.0 Unported, which permits unrestricted use, distribution, and reproduction in any medium, provided the original work is properly cited.
This article was originally published by Medknow Publications & Media Pvt Ltd and was migrated to Scientific Scholar after the change of Publisher.
Abstract
Introduction:
Prepulse inhibition (PPI) is a measurable form of sensorimotor gating. Disruption of PPI reflects the impairment in the neural filtering process of mental functions that are related to the transformation of an external stimuli to a response. Impairment of PPI is reported in neuropsychiatric illnesses such as schizophrenia, Huntington's disease, Parkinson's diseases, Tourette syndrome, obsessive compulsive disorder, and temporal lobe epilepsy with psychosis. Absence epilepsy is the most common type of primary generalized epilepsy. Lamotrigine is an antiepileptic drug that is preferred in absence epilepsy and acts by stabilizing the voltage-gated sodium channels.
Aim:
In this study, we have compared WAG-Rij rats (genetically absence epileptic rats) with Wistar rats, in order to clarify if there is a deficient sensorimotor gating in absence epilepsy, and have examined the effects of lamotrigine (15, 30 mg/kg, i.p.) on this phenomenon.
Materials and Methods:
Depletion in PPI percent value is accepted as a disruption in sensory-motor filtration function. The difference between the Wistar and WAG/Rij rats has been evaluated with the student t test and the effects of lamotrigine on the PPI percent have been evaluated by the analysis of variance (ANOVA) post-hoc Dunnett's test.
Results:
The PPI percent was low in the WAG/Rij rats compared to the controls (P<0.0001, t:9,612). Although the PPI percent value of the control rats was not influenced by lamotrigine, the PPI percent value of the WAG/Rij rats was raised by lamotrigine treatment (P<0.0001, F:861,24).
Conclusions:
As a result of our study, PPI was disrupted in the WAG/Rij rats and this disruption could be reversed by an antiepileptic lamotrigine.
Keywords
Absence epilepsy
lamotrigine
prepulse inhibition
Introduction
Prepulse inhibition (PPI) is a condition in which a weaker prestimulus, which is prepulse, inhibits the reaction of a species to a following strong startling stimulus, which is the pulse. It can be measured in many kinds ranging from rats to humans and the stimuli types are mostly acoustic. The most sensible way to measure PPI is to use muscular reactions, which are normally diminished as a result of the impaired central nervous system functions. When the pulse stimulus is preceded by a weak prepulse stimulus, the motor reflexes can be reduced.[1] Deficits in PPI occur due to the inability of the nervous system to filter out the unnecessary information, and have been linked to abnormalities of sensorimotor gating.[2] Although PPI deficits are mostly seen in schizophrenia, this is not the only situation that may cause such an impairment. PPI has been found to be disrupted in many neuropsychiatric conditions, such as, panic disorder,[3] obsessive-compulsive disorder,[4] Huntington's disease,[5] attention deficit disorder, nocturnal enuresis,[6] and Tourette's syndrome.[7] In this study, the subjects were WAG/Rij (Wistar Albino Glaxo from Rijswijk) rats, which are the widely used genetic model of absence epilepsy. Altough the pathophysiology of absence epilepsy is not fully understood, an abnormally excitable cortex interacted with the thalamus, and brain stem reticular formation is an accepted concept for the formation of absence seizures. The role of the thalamo-cortico-thalamic network in rats, in the occurence of absence seizures, is reported in previous studies.[8] PPI is potently regulated by neural circuitry within the limbic system, frontal cortex, basal ganglia, and pons.[9] Deficits of PPI are not typical to a particular disease, but rather are related to disruptions in certain, yet undefinable brain circuits. In this study, we have compared male WAG-Rij rats (genetically absence epileptic rats) with male Wistar rats, in order to clarify if there is a deficiency in sensorimotor gating in absence epilepsy and examine the effects of lamotrigine (15,30 mg/kg, i.p.) on this phenomenon. Lamotrigine is an anticonvulsant drug used in the treatment of absence epilepsy. Its mechanism of action is based on sodium (Na+)-channel antagonism and inhibition of glutamate release in the brain.[10] Another mechanism of action of lamotrigine is to increase gamma-amino-butyric acid (GABA) release.[11] Lamotrigine may be considered as a treatment option for schizophrenia, as it is known that dysfunctional glutamatergic neurotransmission has a role in the pathophysiology of schizophrenia.[12] It has been seen that lamotrigine, similar to phenytoin,[11] decreases the background release of the major excitatory transmitter glutamate, while increasing the release of the major inhibitory transmitter GABA at synapses in an area of the temporal lobe, which is highly susceptible to epileptogenesis. The combination of these two effects should lead to a powerful idea of excitability in the cortical networks, and may underlie the effectiveness of lamotrigine against partial and generalized seizures. Epilepsy and sensorimotor gating is now yielding to a multifactorial view, in which the monoamines as well as glutamate and GABA are included, with a focus on neurotransmitter interactions in complex neurocircuits.
Materials and Methods
Animals and laboratory
Adult male WAG/Rij rats and adult male Wistar albino rats (Animal Research Center, Kocaeli, Turkey), weighing 200 – 250 g, were housed five to six per cage in an animal colony facility, for two weeks, before the start of the experiment. The animals were maintained in constant room temperature (22±2°C) under a 12-hour light/dark cycle (lights on at 07:00 hours). Tap water and food pellets were provided ad libitum. Each rat was tested only once.
Ethical issue
All procedures complied with the European Community Council Directive of 24 November, 1986, and the Ethics Committee of the Kocaeli University granted the ethical approval (KOU HADYEK 2009, 12/5 - Project Number: 45). The study is original, as it combines a specific kind of animal model of absence epilepsy (WAG/Rij rats) with Wistar rats, using sensorimotor gating phenomenon. There is no conflict of interest.
Prepulse inhibition of startle reaction test apparatus
The tests were conducted using the Animal Acoustic Startle Response System (AASR, Habitest model E10-21, Coulbourn, Pennsylvania, USA) and came through at the same time in two identical startle response systems that were used to record the amplitude of the startle response. The device had special sound proof boxes, with strain gage load cell platforms placed under the animal holders, speakers with a wide sound spectrum, and a computer system to run the software. Each system consisted of a cage suitable for rats weighing 250 – 300 g. Acoustic noise bursts were provided by a loudspeaker located 10 cm behind the cages. The startle reaction of the rat generated a pressure on the response platform and analogical signals were amplified, digitized, and analyzed by a computer software. The sound levels were calibrated using the scale of the sound level meter.
Platform calibration
The cages were calibrated before the test to be sure of the equal sensitivity of both platforms during the sessions. The calibration of platfroms was made by adjusting the gain on the load cell amplifier. We used at least two weights to calibrate the platforms. During platform calibration the mode switch was in the ‘Run/Cal’ position.
Sound calibration
The environment was set to be as conducive as possible, to record the sound pressure level during both calibration and experimental sessions. The sound level meter (Auto range, RS-232) was used to present tone or noise stimuli in order to provide a calibration of amplitude. The background noise level was 62 dB sound pressure level.
Prepulse inhibition session
The prepulse inhibition session was performed following the procedure described previously.[13] First, the rats were placed in the startle cages for a 10-minute adaptation period. After this period, they were presented with a series of five startle pulse-alone trials, followed by a series of five prepulse-alone trials. The pulse-alone trials served only to accommodate the animals to the sudden change in stimulus conditions and were omitted from the data analysis and the prepulse-alone trials were analyzed, only to ensure that these stimuli did not evoke any startle responses on their own. After that, the animals were presented, repeatedly, with a series of five prepulse-pulse trials followed by a series of five pulse-alone trials: Totally 30 trials were conducted. The time between the trials was 10 seconds and the time between any series of trials was 70 seconds. The startle pulse intensity was set at 105 dB. The prepulse intensity was set at 70 dB (8 dB above background noise). The prepulse was 60 milliseconds in duration and presented immediately before the startle pulse, which was 20 milliseconds in duration.
Drugs
All solutions were freshly prepared on the day of testing and administered intraperitoneally (i.p.) in a volume of 0.2 ml per 100 g body weight of the rats. The doses of lamotrigine were chosen based on previous studies. Lamotrigine was a gift from Glaxo Smith Kline, dissolved in 1% acetic acid and warm physiological saline solution.[14] It was administered intraperitoneally at 15 and 30 mg/kg doses. The PPI test was performed 30 minutes after the lamotrigine treatment.[15]
Data analyses
A significant decrease in PPI% was accepted as disruption in the sensory-motor filtration function. In other words it was evaluated as an impairment of the sensory motor gating phenomenon.
The decrease in the amplitude of startle reflex in the presence of prepulse stimulus was calculated according to the formula: PPI% = 100 − (The mean of startle reflex in trials with prepulse) × 100/(The mean of startle reflex in trials without prepulse).
Statistics
Startle amplitude of Wistar and WAG/Rij rats were compared by the student t test. In evaluating the effects of the lamotrigine treatment on startle amplitude (g) of Wistar rats and WAG/Rij rats, one way analyses of variance (ANOVA) and post-hoc Dunnet's test were used. The PPI% of Wistar rats and WAG/Rij rats were compared by the student t test. In evaluating the effects of lamotrigine treatment on PPI% of Wistar rats and WAG/ Rij rats ANOVA and post-hoc Dunnet's test were used. P<0.05 levels were accepted as statistically significant.
Results
Effects of lamotrigine (15, 30 mg/kg) on startle amplitude (g)
The startle amplitude was low in WAG/Rij rats compared to Wistar control rats, (P=0.0002, t:4,86). The startle amplitudes of Wistar rats and WAG/Rij rats were uninfluenced by lamotrigine treatment (P=0,85, F:0,1523) [Figure 1].

- The effects of lamotrigine on the startle amplitude of Wistar rats and WAG/Rij rats (P=0,85, F:0,1523). Each column represents the mean±SEM of 6 – 7 animals.*P=0.0002, compared to the Wistar control (t:4,86)
Effects of lamotrigine (15, 30 mg/kg) on prepulse inhibition (%)
Compaired to Wistar control rats, the PPI% was significantly low in WAG/Rij rats. (P<0.0001, t:9,612). Even as lamotrigine (15, 30 mg/kg) had no effect on the PPI% of Wistar contol rats (P=0.82, F:0,19), the PPI% of lamotrigine-treated (15, 30 mg/kg) WAG/Rij rats showed a significant elevation (P<0.0001, F:861,24) [Figure 2].
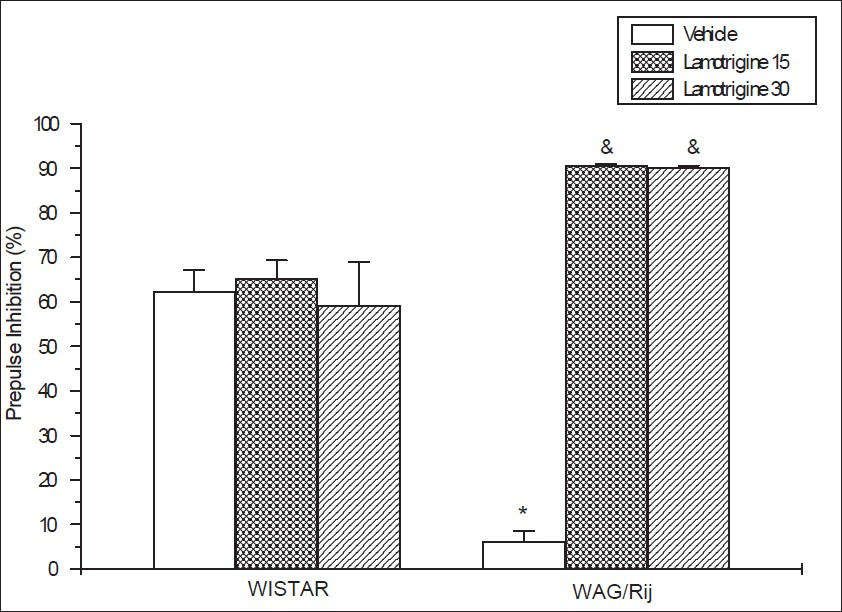
- Effects of lamotrigine on prepulse inhibition% of Wistar rats and WAG/Rij rats. And P<0.0001 (F:861, 24). Each column represents the mean±SEM of 6 – 7 animals. *P<0.0001 compared to Wistar control (t:9,612)
Discussion
Our results demonstrated that, first, the PPI percent value is depleted in WAG/Rij rats compared to Wistar rats, which means that sensorimotor gating is impaired in WAG/Rij rats. Second, lamotrigine treatment (15 mg/ kg and 30 mg/kg) reversed the diminished PPI% of WAG/Rij rats.
This study combines a specific kind of animal model of absence epilepsy (WAG/Rij rats) with naive rats, using the sensorimotor gating phenomenon, to experimentally address the hypothesis that disruption of PPI may develop in WAG/Rij rats. Using the acoustic startle test, we found evidence for the occurrence of PPI impairment in WAG/Rij rats compared to Wistar control rats. Our study has opened a gate to the idea that WAG-Rij rats can be used to investigate biological questions related to the co-morbidity of psychosis and epilepsy.
Clinical disorders with some specific neuroanatomical localizations that address the neural circuit regulation of PPI have been reported in previous studies. In Huntington's disease, diminished PPI may be associated with the degeneration of striatal GABA ergic cells.[5] In Parkinson's disease, the diminished PPI produced by the apomorphine challenge, is suggestive of an enhanced striatal dopamine receptor regulation of PPI.[16] Diminished PPI in patients with temporal lobe epilepsy, with psychosis, and in patients with non-epileptic seizures, suggests some role of the temporal lobe in the regulation of PPI.[17] A study has shown that GABA (B1) heterozygote animals show enhanced PPI responses compared to the controls, suggesting that GABA (B1)-deficient mice exhibit increased sensorimotor gating mechanisms.[18] Absence epilepsy is classified as a generalized seizure type and it has been widely assumed that the thalamus is the generator of this seizure activity. Many studies have been carried out in order to identify the neural circuit regulation of PPI of the startle in rats. In preclinical studies, the brain regions implicated in the regulation of PPI have been probed and defined, for instance, the mediodorsal thalamus[19] and prefrontal cortex[20] are some of the important brain structures. The WAG/Rij albino rat strain is considered to be an appropriate genetic animal model for absence epilepsy.[21] Absence seizures are activated by hyperventilation and occasionally by photic stimulation. Some investigators have concluded that absence seizures are not truly generalized, but rather have selective cortical networks, mainly the ventromedial frontal areas and the somatosensory cortex. The thalamus, especially the reticular nucleus, plays a major role and the frontal cortex, mainly the dorsolateral and orbital frontal areas are important in these seizures.[22] The involvement of the thalamus in limbic epileptogenesis has recently drawn attention to the connectivity between the nuclei of the thalamus and limbic structures. The thalamo-limbic circuits are thought to regulate limbic seizure activity, whereas, the thalamocortical circuits are involved in the expression and generation of spike and slow wave discharges in the absence of epilepsy models. During absence seizures, abnormal rhythms are produced by the interaction between the thalamus and cortex. In a way, the thalamo-cortical circuits have a role in the generation of spike and slow wave discharges in absence epilepsy.[23] The thalamus is an obligatory station through which nearly all sensory information must pass, before reaching the cerebral cortex. One of the major functions of the thalamus is the selective control of the flow of sensory-motor information to the cerebral cortex during different states of the sleep-wake cycle and arousal. This is controlled through the actions of various neurotransmitter systems in the brainstem, hypothalamus, and cerebral cortex.[24] In a similar manner, the limbic and cortico-pallido-striato-thalamic circuitry is thought to be responsible for modulation of PPI in the rat by supporting our findings, and this might be related to impairment of PPI in the absence of epileptic rats. Lamotrigine has the ability to reduce glutamate release, prevent ketamine-induced deficits in PPI in mice, which supports the fact that it exerts its effect through the glutamergic system.[25] The therapeutic effect of lamotrigine on absence seizures may not be related to a main change in a certain neurotransmitter, such as, reduced glutamate, but may have a complex effect that needs to be searched for in further studies. Furthermore, the improvement of PPI impairment via lamotrigine in absence epileptic rats may be related to the thalamocortical system, which is a probable therapeutic target of this anticonvulsant drug.[26] This suggests that lamotrigine, an anticonvulsant, mood stabilizer, may have an undefinable affect, beyond stabilizing voltage-sensitive sodium channels, on absence epileptic rats that could make it effective in PPI impairment. The results of this study demonstrate that PPI disrupted in WAG/Rij rats and this disruption could be reversed by lamotrigine. This at least, in part, may be used to investigate the comorbidity of psychosis and epilepsy.
We would like to gratefully and sincerely thank Prof. Nurbay Ates for his guidance and contribution.
Source of Support: Nil
Conflict of Interest: None declared.
References
- Pharmacological studies of prepulse inhibition models of sensorimotor gating deficits in schizophrenia: A decade in review. Psychopharmacology. 2001;156:117-54.
- [Google Scholar]
- Human studies of prepulse inhibition of startle: Normal subjects, patient groups, and pharmacological studies. Psychopharmacology. 2001;156:234-58.
- [Google Scholar]
- Information-processing deficits and cognitive dysfunction in panic disorder. Psychiatry Neurosci. 2005;30:37-43.
- [Google Scholar]
- Impaired prepulse inhibition of acoustic startle in obsessive-compulsive disorder. Biol Psychiatry. 2005;57:1153-8.
- [Google Scholar]
- Impaired prepulse inhibition of acoustic and tactile startle response in patients with Huntington's disease. J Neurol Neurosurg Psychiatry. 1995;58:192-200.
- [Google Scholar]
- Prepulse inhibition of startle, intelligence and familial primary nocturnal enuresis. Acta Paediatr. 2000;89:475-81.
- [Google Scholar]
- Sensorimotor gating in boys with Tourette's syndrome and ADHD: Preliminary results. Biol Psychiatry. 1996;39:33-41.
- [Google Scholar]
- On the cellular and network basis of epileptic seizures. Annu Rev Physiol. 2001;63:815-46.
- [Google Scholar]
- Neural circuit regulation of prepulse inhibition of startle in the rat: Current knowledge and future challenges. Psychopharmacology. 2001;156:194-215.
- [Google Scholar]
- The anticonvulsant, lamotrigine decreases spontaneous glutamate release but increases spontaneous GABA release in the rat entorhinal cortex in vitro. Neuropharmacology. 2000;39:2139-46.
- [Google Scholar]
- The emerging role of glutamate in the pathophysiology and treatment of schizophrenia. Am J Psychiatry. 2001;158:1367-77.
- [Google Scholar]
- Effects of phencyclidine on acoustic startle and prepulse inhibition in neuronal nitric oxide synthase deficient mice. Eur Neuropsychopharmacol. 2005;15:587-90.
- [Google Scholar]
- Studies on induction of lamotrigine metabolism in transgenic UGT1 mice. Xenobiotica. 2009;39:826-35.
- [Google Scholar]
- Mood stabilizers increase prepulse inhibition in DBA/2NCrl mice. Psychopharmacology (Berl). 2009;205:369-77.
- [Google Scholar]
- The effects of apomorphine and L-dopa challenge on prepulse inhibition in patients with Parkinson's disease. Schizophr Res. 1995;15:181.
- [Google Scholar]
- Epileptogenesis and enhanced prepulse inhibition in GABA(B1)-deficient mice. Mol Cell Neurosci. 2001;17:1059-70.
- [Google Scholar]
- Regulation of prepulse inhibition by ventral pallidal projections. Brain Res Bull. 1997;43:219-28.
- [Google Scholar]
- Deficient sensorimotor gating after 6-hydroxydopamine lesion of the rat medial prefrontal cortex is reversed by haloperidol. Eur J Neurosci. 1994;6:1837-45.
- [Google Scholar]
- Genetic animal models for absence epilepsy: A review of the WAG/Rij strain of rats. Behav Genet. 2003;33:635-55.
- [Google Scholar]
- Absence seizures: A review of recent reports with new concepts. Epilepsy Behav. 2009;15:404-12.
- [Google Scholar]
- Lamotrigine prevents ketamine but not amphetamine-induced deficits in prepulse inhibition in mice. Psychopharmacology (Berl). 2003;169:240-6.
- [Google Scholar]
- Anticonvulsant actions of lamotrigine on spontaneous thalamocortical rhythms. Epilepsia. 2002;43:342-9.
- [Google Scholar]