Translate this page into:
Transcranial direct current stimulation-efficacy in mild cognitive impairment: A meta-analysis
*Corresponding author: Thomas Gregor Issac, Centre for Brain Research, Indian Institute of Science, Bengaluru, Karnataka, India. thomasgregor@iisc.ac.in
-
Received: ,
Accepted: ,
How to cite this article: Sandhya G, Malo P, Issac TG. Transcranial direct current stimulation-efficacy in mild cognitive impairment: A meta-analysis. J Neurosci Rural Pract. 2024;15:194-202. doi: 10.25259/JNRP_629_2023
Abstract
Objectives:
Mild cognitive impairment (MCI) is a transition state in which individuals have cognitive abilities that are in between those of normal aging and dementia. Although not everyone with MCI develops dementia, the risk of progression to dementia is higher in people with MCI. Interventions at this stage can prevent or delay the onset of dementia. In recent years, studies on non-invasive brain stimulation techniques, namely transcranial direct current stimulation (tDCS), have gained momentum for cognitive enhancement in MCI. Since there are very few studies that also report varied results, it becomes important to analyze the effect of tDCS in MCI. The aim of this study was to systematically review the available evidence about using tDCS for MCI and to assess its efficacy using meta-analysis.
Materials and Methods:
Eight single- or double-blinded randomized controlled trials were included in the study. Montreal cognitive assessment (MoCA) and mini-mental state examination (MMSE) for global cognition; and digit span test forward and backward, trail-making test (TMT) A and B; and logical memory test (LMT) assessing specific cognitive domains were considered. A random-effects model was used wherein the standardized mean difference (SMD) and its 95% confidence intervals were reported.
Results:
The effect of the active tDCS (MoCA [SMD 0.37, 95% CI −0.22–0.95], MMSE [SMD 0.26, 95% CI 0.25–0.77], TMT-A [SMD −0.01, 95% CI −0.42–0.40], and LMT [SMD 0.80, 95% CI −0.24–1.83]) when compared with the sham tDCS was statistically insignificant.
Conclusion:
The current meta-analysis identified insignificant improvement in cognitive performance with active tDCS treatment as compared to sham tDCS among people with MCI.
Keywords
Transcranial direct current stimulation
Mild cognitive impairment
Cognition
INTRODUCTION
Neurodegenerative diseases are a cause of severe disability and mortality. It is seen that the burden of these diseases including dementia has been increasing in recent times and is expected to increase more extensively in further years.[1] Mild cognitive impairment (MCI) is a state in which a person’s cognitive abilities are in between those of normal and dementia-affected people. This stage is considered a prodromal stage of dementia. Although not all people with MCI certainly progress to dementia,[2] it is seen that people with MCI have a higher risk of developing dementia than others.[3] A prospective cohort study in Italy by Ravaglia et al., in 2008, observed a 14% conversion rate from MCI to dementia in a year.[4] Most cohort studies identified rates of conversion between 10% and 15% annually.[2] Due to the high risk and unavailability of disease-modifying therapy for dementia, there has been increased attention in recent years on introducing interventions at the MCI stage to prevent or delay the progression to dementia.
The common interventions are cognitive training based, which focus on different cognitive domains. Physical activity and socializing were also found to be effective interventions for MCI.[5] Other interventions include non-invasive brain stimulation (NIBS) techniques. Most common NIBS include transcranial direct current stimulation (tDCS),[6] transcranial alternating current stimulation,[7] and repetitive transcranial magnetic stimulation.[8] These were initially used as interventions for people with dementia but are now studied as potential interventions for the preclinical stage. Among these, tDCS is an emerging neuromodulatory therapy wherein direct current is delivered to particular brain regions of interest. The current is not sufficient to induce action potentials in neurons but increases or decreases the susceptibility of the neurons to get activated. If an anode is placed on the region of interest, the region gets activated while a cathode causes inhibition of the region. Anodal tDCS, which stimulates the region of interest, has been found to be effective in treating depression,[9] anxiety,[10] and also cognitive decline,[11] especially when coupled with other nonpharmacological interventions.
Several studies on patients with Alzheimer’s dementia[12] and other types of dementia[13,14] using anodal tDCS found significant improvements in cognitive performance after treatment compared to patients undergoing sham treatment. There are a handful of studies looking at the effect of anodal tDCS administration on cognitive functioning in people with MCI.[15]
The studies using tDCS on people with MCI did not identify improvements in all tests of different cognitive domains.[16] There is mixed evidence and a lack of understanding of the utility of tDCS as an intervention in dementia and also in MCI. Hence, it becomes important to review such studies to look at the effectiveness of this type of non-invasive treatment. Published meta-analyses on the utility of tDCS for cognitive impairment considered people with MCI as well as people with early/mid dementia.[17] The effectiveness of tDCS intervention has not been analyzed exclusively on people with MCI. Thus, the present study aims to check the efficacy of active tDCS in improving cognitive functioning in people with MCI when compared with those who receive sham stimulation.
MATERIALS AND METHODS
The present study was restricted to publications in the English language. Since the majority of the publications are cited in Google Scholar, it was used as one of the search engines for the review along with PubMed Central. The search term, ‘(“tDCS”) AND (“MCI”) AND (Randomized) AND (sham controlled)’ was used, and 221 citations from PubMed Central and 686 citations from Google Scholar were obtained. Studies published before November 2022 were included in the study. From the total of 907 results, 225 results were removed due to duplication. Then, articles other than original research were excluded from the study. Among the original articles, the records obtained were further filtered based on certain criteria. The inclusion criteria consist of (1) studies including people with MCI diagnosis; (2) studies using tDCS OR high definition tDCS (HD-tDCS) as an intervention either alone or along with other interventions; (3) studies having a group, who receive sham tDCS with or without other interventions; (4) studies measuring cognition as one of their outcomes; and (5) single- or double-blinded randomized controlled trials (RCTs). The exclusion criteria comprised (1) studies with a diagnosis of any type of dementia; (2) studies without a sham group; (3) studies whose outcome variable is not any standard cognitive test; and (4) studies on animal models.
A total of 17 articles were shortlisted. Among these, three articles did not have data for any standard cognitive tests. From the remaining 14 studies, the neuropsychological tests that were performed in at least three of the studies were considered for the review and analysis. Eight tests including mini-mental state examination (MMSE), Montreal cognitive assessment (MoCA), digit span test forward and backward (DST F and B), trail-making tests A and B (TMT A and B), and logical memory test (LMT) delayed recall were considered. MoCA is a cognitive screening test, which tests for orientation, naming, memory, attention, abstraction, language, visuospatial, and executive functioning. The MMSE is also a cognitive screening test, which takes less time to administer than MoCA. It contains tests for orientation, registration, recall, attention, language, and praxis.
The TMT-A is a test for working memory and involves the joining of numbers in ascending order. The TMT-B is a test for executive functioning and involves joining numbers and letters alternatingly in consecutive order. In DST-F, the participant is asked to repeat a series of numbers after listening. This task primarily tests attention. In DST-B, the participant is asked to repeat a series of numbers in the reverse order after listening to the number. This is a test for working memory. The LMT is a test for episodic memory wherein the participants are made to listen to a story after which they are asked to recall the story immediately and then 20–30 min later. For the current analysis, the delayed recall of the LMT was considered.
Finally, eight articles, which had data for the above-mentioned seven tests were included in the meta-analysis.[16,18-24] From these studies, the pre- and post-intervention scores in various cognitive tests were extracted and then analyzed. Apart from the scores, information about sample size in each study group, mean age, site of stimulation, duration and intensity of tDCS, frequency of intervention, and details of any adjuvant training were extracted. Two authors (SG and TGI) reviewed the search results independently, verified the study’s eligibility, and extracted the data. The extracted data was again independently verified by another author (PKM). The protocol was registered with PROSPERO [CRD42023474376]. The search flowchart has been provided in the supplementary material [Supplementary Figure 1], and the characteristics of eight studies are provided in Table 1. The Preferred Reporting Items for Systematic Reviews and Meta-Analyses checklist is provided in Supplementary Table 1.
Sl. No. | Author | Year of publication | Population | Age | Sample size | Intervention | Site of stimulation | Intensity | Duration | Number of sessions | Training | Outcomes included |
---|---|---|---|---|---|---|---|---|---|---|---|---|
1. | Gonzalez et al. | 2021 | MCI | 69.8±5.3 and 71.0±6.2 | 22 vs. 24 | atDCS | Left DLPFC | 1.5mA | 30 min | 9 sessions (3 weeks) | Working memory and attention tasks | MoCA, DST - F and B, TMT - A and B |
2. | He et al. | 2021 | MCI | 63.5±2.8 and 65.63±3.53 | 24 vs. 19 | HD tDCS | Left DLPFC | 1.0mA | 20 min | 10 sessions (2 weeks) | No training | MMSE, MoCA |
3. | Aksu et al. |
2022 | PD-MCI | 65.52±7.49 | 13 vs. 13 | atDCS | Left DLPFC | 2.0mA | 20 min | 10 sessions (5 days) | No training | TMT - A, DST - F and B, LMT |
4. | Lu et al. | 2019 | Mild NCD-AD | 74.2±6.7 and 74.5±6.6 | 69 vs. 64 | atDCS | Left lateral temporal cortex | 2.0mA | 20 min | 12 sessions (4 weeks) | Adaptive N-back test - Working memory training (WMT) | LMT, DST - F |
5. | Liao et al. | 2021 | MCI | 72.6±4.1 and 73.1±4.6 | 11 vs. 10 | atDCS | Left DLPFC | 2.0mA | 20 min | 36 sessions (12 weeks) | Tai Chi | MoCA, TMT - A and B |
6. | Gu et al. | 2022 | MCI | 63.20±6.98 and 65.15±6.16 | 20 vs. 20 | atDCS | Left temporal cortex | 2.0mA | 20 min | 5 sessions (5 days) | No training | MoCA, LMT |
7. | Zhang et al. |
2022 | aMCI | 57.11±3.10 and 56.92±2.78 | 35 | HD tDCS | Left DLPFC | 1.0mA | 20 min | 10 sessions (2 weeks) | No training | MMSE, MoCA |
8. | Gomes et al. | 2019 | MCI | 73.0±9.2 and 71.6±7.9 | 29 vs. 29 | atDCS | Left DLPFC | 2.0mA | 30 min | 10 sessions (5 weeks) | No training | MMSE, DST - F and B |
DLPFC: Dorsolateral pre-frontal cortex, MoCA: Montreal cognitive assessment, MMSE: Mini-mental state examination, TMT-A: Trail-making test A, TMT-B: Trail-making test B, LMT: Logical memory test, DST-F: Digit span test forward, DST-B: Digit span test backward, MCI: Mild cognitive impairment, PD-MCI: Parkinson’s disease - mild cognitive impairment, NCD-AD: Neurocognitive disorder - Alzheimer’s disease, aMCI: Amnestic mild cognitive impairment, atDCS: Anodal transcranial direct current stimulation, HD tDCS: High definition transcranial direct current stimulation.
Meta-analysis
Eight studies[16,18-24] were assessed using the PICO (Population, Intervention, Comparison, and Outcomes) format recommended by the Cochrane Collaboration and were found eligible to include in a meta-analysis. Hedge’s g standardized mean differences (SMDs) and its 95% confidence intervals (CIs) were calculated for MoCA,[19-21,23,24] MMSE,[16,21,24] TMT-A,[19,20,22] TMT-B,[19,20] DST-F,[16,18,20,22] DST-B,[16,20,22] and LMT[18,22,23] using inverse-variance-based DerSimonian and Laird’s estimation method. We used a random effects model to estimate SMDs and its CIs. We adopted intention-to-treat analysis wherein the total sample size was randomized for the intervention, and the placebo groups at the beginning of the study were considered instead of completers. Analyses were performed using Cochrane RevMan 5.3. Finally, the statistical significance was checked at a 5% level of significance, and the impact of heterogeneity was measured by I2-statistic.
Subgroup analysis
Since tDCS protocols have not yet been standardized, there exists a lot of heterogeneity among the studies. Data on MoCA scores was available for five studies. Hence, MoCA scores were used for the subgroup analyses. We performed three subgroup analyses. The studies were divided based on the (a) sample size > 30 or ≤ 30, (b) stimulation intensity <2.0 mA or ≥2.0 mA, and (c) whether any kind of adjuvant training was given or not.
Sensitivity analysis
To study the effect after excluding studies with extreme parameters, five sensitivity analyses were performed. Again, MoCA scores were used for the sensitivity analyses. The analyses were done after excluding studies that had a mean age of >70 years, studies that used HD-tDCS as the intervention, studies whose duration was longer than three weeks, studies that did not use left dorsolateral prefrontal cortex as the stimulation site, and studies which used a stimulation duration of 30 min.
Risk of bias assessment
The included studies were assessed for the risk of bias using the Cochrane risk of bias tool. It was evaluated for the following seven domains: (1) Random sequence generation; (2) allocation concealment; (3) blinding of participant and personnel; (4) blinding of outcome assessment; (5) incomplete outcome data; (6) selective reporting; and (7) other bias. The domains were reported as “low risk,” “high risk,” and “unclear risk.”
RESULTS
Eight studies were included in the study based on the selection criteria and cognitive assessments used. The studies used 1–2 mA direct current for the interventions. The number of tDCS sessions varied between 5 and 36 that spanned a period of 5 days to 12 weeks. Some studies also had some form of cognitive training[18-20] given along with anodal tDCS intervention.
Primary outcomes
MoCA
Among the eight studies, a total of five studies had data about MoCA scores of MCI people, who underwent anodal tDCS and sham tDCS.[19-21,23,24] A total of 94 people were in the treatment group, and a total of 86 people were in the sham group. The pooled SMD and its 95% CI was 0.37 (−0.22, 0.95). It indicated that the difference in the performance of the active tDCS group was not statistically significant when compared with the sham group (z = 1.23; P = 0.22) with I2 = 72% (χ2 = 14.48; P = 0.006) [Figure 1a].

- (a) Forest plot for studies with MoCA scores, (b) Forest plot for studies with MMSE scores. MMSE: Mini-mental state examination, tDCS: Transcranial direct current stimulation, SD: Standard deviation, CI: Confidence interval, Position of the green box: Standardized mean difference, Size of the green box: Study weight, MoCA: Montreal cognitive assessment.
MMSE
Three studies used MMSE as one of the outcome variables.[16,21,24] A total of 70 and 61 people with MCI in treatment and sham groups, respectively, were considered for the analysis. The pooled SMD (95% CI) obtained was 0.26 (−0.25, 0.77) and denoted that the difference in MMSE scores among the two groups was insignificant (z = 1.00; P = 0.32) with I2 = 51% (χ2 = 4.09; P = 0.13) [Figure 1b].
Secondary outcomes
TMT-A
There was a total of four studies that included TMT-A data.[19,20,22] The mean value for this test is time in seconds. However, in one of the studies, namely, Gomes, the unit for a time was not mentioned, and the values were in a very different range.[16] Hence, the study was not included in the TMT-A analysis. Overall, the analysis includes data from 46 participants from the treatment group and 47 from the sham group. Since the variable here is time and a shorter time for completion of the test denotes better performance, a negative effect means that there is favoring of anodal tDCS. From the overall SMD and its 95% CI, −0.01 (−0.42, 0.40), it was evident that the difference in performance was insignificant (z = 0.06; P = 0.95) with I2 = 0% (χ2 = 1.63; P = 0.44) [Figure 2a].
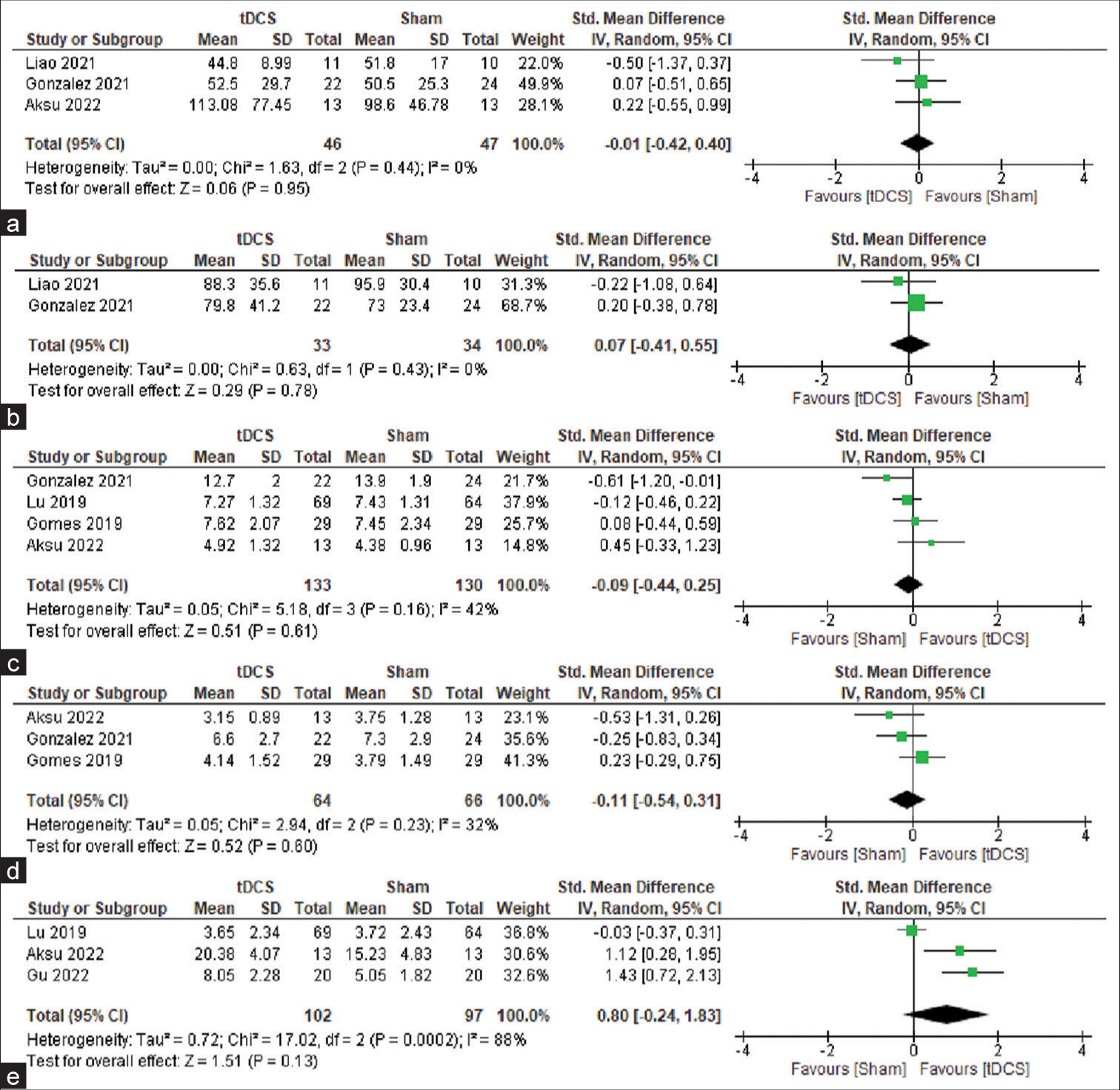
- (a) Forest plot for studies with TMT-A scores, (b) Forest plot for studies with TMT-B scores, (c) Forest plot for studies with DST-F scores, (d) Forest plot for studies with DST-B scores, and (e) Forest plot for studies with LMT-DR scores. TMT-A: Trail-making test A, TMT-B: Trail-making test B, LMT: logical memory test, DST-F: Digit span test forward, DST-B: Digit span test backward. tDCS: Transcranial direct current stimulation, SD: Standard deviation, CI: Confidence interval, Position of the green box: Standardized mean difference, Size of the green box: Study weight.
TMT-B
Three papers had data for TMT-B.[19,20] Since Gomes had been excluded from the TMT-A analysis, it was also excluded from the TMT-B analysis.[16] In the two studies included, there were 33 and 34 people in total for the intervention and sham groups, respectively. Here also, the mean values indicate the time required to complete the test. Hence, an overall SMD and its 95% CI of 0.07 (−0.41, 0.55) denoted an insignificant difference in TMT-B scores in the active tDCS group when compared with the sham tDCS group (z = 0.29; P = 0.78) with I2 = 0% (χ2 = 0.63; P = 0.43) [Figure 2b].
DST-F
A total of four studies that have pre- and post-treatment scores were included in the analysis.[16,18,20,22] This included data from 133 participants, who underwent active tDCS and 130 participants, who underwent sham tDCS. The overall SMD (95% CI) obtained was −0.09 (−0.44, 0.25), which implied that the difference in DST-F scores was not significant among the two groups (z = 0.51; P = 061) with I2 = 42% (χ2 = 5.18; P = 0.16) [Figure 2c].
DST-B
Among the studies that were selected based on the criteria, three had data for DST-B.[16,20,22] These included 64 people with MCI people in the active tDCS group and 66 people with MCI in the sham tDCS group. The pooled SMD and its 95% CI obtained overall is −0.11 (−0.54, 0.31). This result implied that the difference between the two groups in DST-B was insignificant (z = 0.52; P = 0.60) with I2 = 32% (χ2 = 2.94; P = 0.23) [Figure 2d].
LMT-DR
A total of three studies with 102 people in the active tDCS group and 97 people in the sham tDCS group were included in the current meta-analysis.[18,22,23] The overall SMD and 95% CI of 0.80 (−0.24, 1.83) suggested that the difference in performance in LMT-DR was insignificant (z = 1.51; P = 0.13) with I2 = 88% (χ2 = 17.02; P < 0.001) [Figure 2e].
Subgroup analysis
Three subgroup analyses were done to reduce the effect of variability among the various studies.
In the first group analysis, the studies were classified based on the sample size into two groups namely ≤ 30 and >30. In the first group consisting of studies with ≤30 samples each, there were two studies.[19,24] The overall SMD was 0.83 with a 95% CI −1.02–2.68. Thus, among the studies that had smaller sample sizes, the difference in MoCA scores among the groups was insignificant (z = 0.88; P = 0.38) with I2 = 89% (χ2 = 9.18; P = 0.002). The second group included studies with a sample of more than 30. There were three studies that satisfied this criterion and had MoCA scores.[20,21,23] The overall SMD and the 95% CI are 0.11 (−0.24, 0.46). This suggested that the difference in performances is not statistically significant (z = 0.63; P = 0.53) with I2 = 0% (χ2 = 1.40; P = 0.50) [Supplementary Figures 2a and b].
Another subgroup analysis was done, and the basis of classification was the intensity of current given during active anodal tDCS. According to this grouping, the first subgroup included three studies, which used a current intensity of less than 2.0mA.[20,21,24] The overall SMD (95% CI) of 0.52 (−0.48, 1.53) suggested an insignificant difference in MoCA scores among the two groups (z = 1.02; P = 0.31) with I2 =85% (χ2 = 13.44; P = 0.001). The second group consisted of two studies, which used a 2.0 mA current for the active stimulation.[19,23] The two studies have an overall SMD and 95% CI of 0.24 and −0.27–0.75, respectively. From this, it can be inferred that the difference among MoCA scores is insignificant (z = 0.92; P = 0.36) with I2 = 0% (χ2 = 0.99; P = 0.32) [Supplementary Figures 3a and b].
The last subgroup analysis was performed to check if giving any adjuvant therapy influences the effectiveness of tDCS in MCI. The first group included three studies, which did not include any adjuvant training.[21,23,24] The studies have a pooled SMD (95% CI) of 0.68 (−0.26, 1.62). This implied that the difference in MoCA scores between the two groups was not significant (z = 1.42; P = 0.16) with I2 = 82% (χ2 = 11.12; P = 0.004). The other subgroup, which does not involve any adjuvant training, had two studies with MoCA scores.[19,20] The overall SMD and its 95% CI of −0.06 and −0.54 to 0.42 indicated that the difference observed in MoCA scores among the two groups was not significant statistically (z = 0.26; P = 0.80) with I2 = 0% (χ2 = 0.02; P = 0.89) [Supplementary Figures 4a and b].
Sensitivity analysis
Five sensitivity analyses were made based on the MoCA scores of participants, who underwent active and sham tDCS post-intervention.
In the first analysis, studies that had participants with a mean age of more than 70 years were excluded from the study. Three studies remained.[21,23,24] The overall SMD and its 95% CI was 0.58 and (−0.26, 1.62) which implied that the difference in performances among the two groups was not statistically significant (z = 1.42; P = 0.16) with I2 = 82% (χ2 = 11.12; P = 0.004) [Supplementary Figure 5].
A second analysis was made excluding the studies that used HD-tDCS as the intervention. This left us with three studies, which used conventional anodal as the intervention.[19,20,23] The overall SMD (95% CI) between active tDCS and sham tDCS group was 0.12 (−0.26, 0.50). This implied no significant effect of active tDCS (z = 0.61; P = 0.54) with I2 = 0% (χ2 = 1.49; P = 0.47) [Supplementary Figure 6].
The next sensitivity analysis excluded studies that gave the intervention for more than three weeks. There were four studies that remained.[20,21,23,24] The overall SMD (95% CI) of 0.48 (−0.22, 1.18) suggested a non-significant difference in MoCA scores among the two groups (z = 1.35; P = 0.18) with I2 = 78% (χ2 = 13.54; P = 0.004) [Supplementary Figure 7].
In the next sensitivity analysis, a study wherein the stimulation site was the left temporal cortex and not the left dorsolateral prefrontal cortex was excluded from the study. Four studies remained.[19-21,24] These had an overall SMD (95% CI) of 0.36 (−0.41, 1.14). This suggested a non-significant difference in MoCA scores among the two groups (z = 0.93; P = 0.35) with I2 = 79% (χ2 = 14.23; P = 0.003) [Supplementary Figure 8].
Four studies had a stimulation duration of 20 min/session.[19,21,23,24] The one study, which had a stimulation duration of 30 min, was excluded to perform the present sensitivity analysis.[20] An overall SMD (95% CI) of 0.49 (−0.26, 1.24) implied that the difference between anodal tDCS and sham tDCS groups was not significant (z = 1.28; P = 0.20) with I2 = 77% (χ2 = 12.82; P = 0.005) [Supplementary Figure 9].
Risk of bias assessment
There was no risk of performance, detection, or attrition biases; but had a high risk of selection bias. Other risks were unknown [Figure 3].
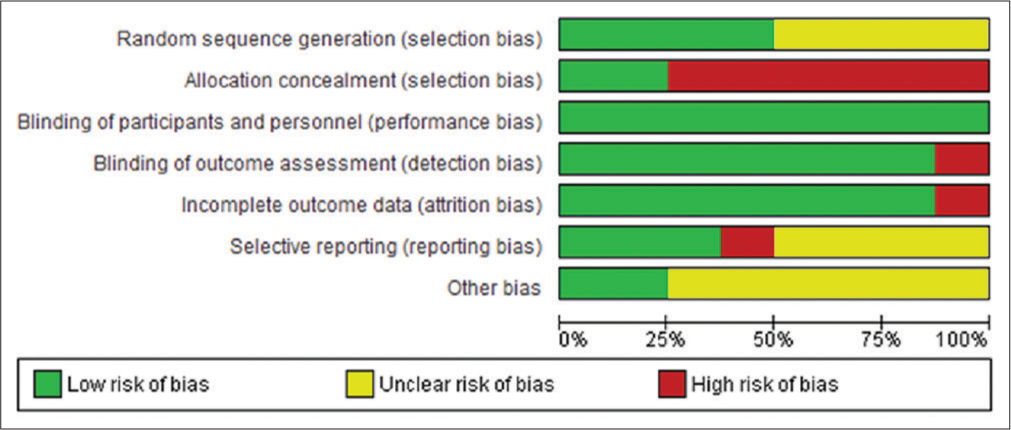
- Risk of bias across all included studies.
DISCUSSION
The utility of tDCS for cognitive enhancement has been studied in recent years, especially in dementia. The tDCS intervention in people with MCI has not been extensively studied. From the available evidence, the present meta-analysis identified no significant improvement in performance in any of the cognitive tests considered. Considering the studies individually, it was seen that the domains of cognition that were improved with tDCS in MCI most commonly were memory and executive functioning. Most of the studies do not show significant differences in global cognition. The study by Zhang et al. showed a significant difference in MoCA scores in the treated group compared with the sham tDCS group.[24] This result was extremely different when compared to other studies. One possible reason for this might be the lower mean age of participants included in the study (active tDCS – 57.11 years and sham tDCS – 56.92 years).
Although there were differences in performance of various cognitive tests, none of the differences were significant among the two groups. The previous meta-analyses on using tDCS to improve cognitive functioning observed significant improvements in people with dementia than in people with MCI.[17] The present study, which used data from MCI patients only, also did not find significance in the improvements after treatment. One possible reason may be that the cognitive symptoms in MCI are very mild, and thus, the improvement was not evident. Furthermore, the evaluation scales used might not be sensitive enough to capture small changes in cognitive functioning among people with MCI.
The strength of this study was that it did not include people with mild or moderate dementia. Since many meta-analyses took study participants with MCI and mild dementia together, the effect on MCI patients alone could not be isolated. The present study was efficient in doing so. One of the limitations was the availability of a handful of independent studies with inadequate sample size, which did not result in enough overall sample size. Furthermore, the heterogeneity among the studies was very high. The tDCS protocol used in different studies varied widely. The development of a standard protocol for tDCS intervention would make the results of the analysis clearer. Since tDCS uses mild modulation of brain regions to produce the effect, a higher number of sessions are required to see clear effects. The number of sessions did not exceed 12 in all but one study[19] while the intensity used was less than the commonly used intensity of 2.0 mA in many studies. Furthermore, most of the studies used other interventions of cognitive training or physical exercise along with tDCS whose effect might have interfered with the action of tDCS.
To minimize the above effects, future studies may focus on a larger sample size. Moreover, data about the long-term effects rather than immediate benefits of the intervention would be more valuable to the field of aging. For this purpose, RCTs with multiple follow-ups after the end of the tDCS treatment are required. These can help in identifying the age of onset of dementia, which might be a better marker for the effect of tDCS. This could also overcome the limitation of cognitive tests not being able to identify minor changes in cognitive performance among people with MCI.
CONCLUSION
The present study found that administering active tDCS improved performance in tests for global cognition (MCA and MMSE) as well as assessments specific to attention (TMT-A) and memory (LMT) domains. However, these differences between the active and sham tDCS groups were not statistically significant. Further studies with standardized protocols and larger sample sizes may be required to fully assess the efficacy of tDCS in improving cognitive function in people with MCI.
Acknowledgment
We would like to acknowledge the Director of the institute for facilitating the study.
Authors’ contributions
TGI formulated the research question, designed the study, supervised data acquisition, and was involved in manuscript preparation and editing. PKM designed the study, supervised data acquisition, analyzed the data, and prepared the manuscript and editing. SG was involved in the literature search, data acquisition, manuscript preparation, and editing.
Ethical approval
The Institutional Review Board approval is not required.
Declaration of patient consent
Patient’s consent not required as there are no patients in this study.
Conflicts of interest
There are no conflicts of interest.
Use of artificial intelligence (AI)-assisted technology for manuscript preparation
The authors confirm that there was no use of artificial intelligence (AI)-assisted technology for assisting in the writing or editing of the manuscript and no images were manipulated using AI.
Financial support and sponsorship
Nil.
References
- Blueprint for dementia research. 2022. :72. Available from: https://www.who.int/publications/i/item/9789240058248 [Last accessed on 2023 Sep 20]
- [Google Scholar]
- Classification and epidemiology of MCI. Clin Geriatr Med. 2013;29:753-72.
- [CrossRef] [PubMed] [Google Scholar]
- Risk factors for the progression of mild cognitive impairment to dementia. Clin Geriatr Med. 2013;29:873-93.
- [CrossRef] [PubMed] [Google Scholar]
- Mild cognitive impairment: Epidemiology and dementia risk in an elderly Italian Population. J Am Geriatr Soc. 2008;56:51-8.
- [CrossRef] [PubMed] [Google Scholar]
- Systematic review of strengths and limitations of randomized controlled trials for non-pharmacological interventions in mild cognitive impairment: Focus on Alzheimer's disease. J Nutr Health Aging. 2015;19:141-53.
- [CrossRef] [PubMed] [Google Scholar]
- Using transcranial direct current stimulation to treat symptoms in mild cognitive impairment and Alzheimer's disease. Neurodegener Dis Manag. 2017;7:317-29.
- [CrossRef] [PubMed] [Google Scholar]
- The impact of gamma transcranial alternating current stimulation (tACS) on cognitive and memory processes in patients with mild cognitive impairment or Alzheimer's disease: A literature review. Brain Stimul. 2023;16:748-55.
- [CrossRef] [PubMed] [Google Scholar]
- Transcranial magnetic stimulation (TMS)/repetitive TMS in mild cognitive impairment and Alzheimer's disease. Acta Neurol Scand. 2014;129:351-66.
- [CrossRef] [PubMed] [Google Scholar]
- A review of transcranial direct current stimulation (tDCS) for the individualized treatment of depressive symptoms. Pers Med Psychiatry. 2019;17-18:17-22.
- [CrossRef] [PubMed] [Google Scholar]
- Transcranial direct current stimulation in patients with anxiety: Current perspectives. Neuropsychiatr Dis Treat. 2020;16:161-9.
- [CrossRef] [PubMed] [Google Scholar]
- New methods, old brains-a systematic review on the effects of tDCS on the cognition of elderly people. Front Hum Neurosci. 2021;15:730134.
- [CrossRef] [PubMed] [Google Scholar]
- Role of transcranial direct current stimulation in the management of Alzheimer's disease: A meta-analysis of effects, adherence and adverse effects. Clin Psychopharmacol Neurosci. 2021;19:589-99.
- [CrossRef] [PubMed] [Google Scholar]
- Effects of tDCS on executive function in Parkinson's disease. Neurosci Lett. 2014;582:27-31.
- [CrossRef] [PubMed] [Google Scholar]
- Transcranial stimulation in frontotemporal dementia: A randomized, double-blind, sham-controlled trial. Alzheimer's and dementia. Transl Res Clin Interventions. 2020;6:e12033.
- [CrossRef] [PubMed] [Google Scholar]
- Can Transcranial direct-current stimulation alone or combined with cognitive training be used as a clinical intervention to improve cognitive functioning in persons with mild cognitive impairment and dementia? A systematic review and meta-analysis. Front Hum Neurosci. 2018;12:416.
- [CrossRef] [PubMed] [Google Scholar]
- Transcranial direct current stimulation (tDCS) in elderly with mild cognitive impairment: A pilot study. Dement Neuropsychol. 2019;13:187-95.
- [CrossRef] [PubMed] [Google Scholar]
- Transcranial direct current stimulation enhances cognitive function in patients with mild cognitive impairment and early/mid Alzheimer's disease: A systematic review and meta-analysis. Brain Sci. 2022;12:562.
- [CrossRef] [PubMed] [Google Scholar]
- Randomized controlled trial of TDCS on cognition in 201 seniors with mild neurocognitive disorder. Ann Clin Transl Neurol. 2019;6:1938-48.
- [CrossRef] [PubMed] [Google Scholar]
- Combining transcranial direct current stimulation with tai chi to improve dual-task gait performance in older adults with mild cognitive impairment: A randomized controlled trial. Front Aging Neurosci. 2021;13:766649.
- [CrossRef] [PubMed] [Google Scholar]
- Transcranial direct current stimulation as an adjunct to cognitive training for older adults with mild cognitive impairment: A randomized controlled trial. Ann Phys Rehabil Med. 2021;64:101536.
- [CrossRef] [PubMed] [Google Scholar]
- Repeated anodal high-definition transcranial direct current stimulation over the left dorsolateral prefrontal cortex in mild cognitive impairment patients increased regional homogeneity in multiple brain regions. PLoS One. 2021;16:e0256100.
- [CrossRef] [PubMed] [Google Scholar]
- Does transcranial direct current stimulation enhance cognitive performance in Parkinson's disease mild cognitive impairment? An event-related potentials and neuropsychological assessment study. Neurol Sci. 2022;43:4029-44.
- [CrossRef] [PubMed] [Google Scholar]
- The effect and mechanism of transcranial direct current stimulation on episodic memory in patients With mild cognitive impairment. Front Neurosci. 2022;16:811403.
- [CrossRef] [PubMed] [Google Scholar]
- Repeated high-definition transcranial direct current stimulation modulated temporal variability of brain regions in core neurocognitive networks over the left dorsolateral prefrontal cortex in mild cognitive impairment patients. J Alzheimers Dis. 2022;90:655-66.
- [CrossRef] [PubMed] [Google Scholar]