Translate this page into:
Pattern Reversal Visual Evoked Potential and Cognitive Functions in Subclinical Hypothyroid Subjects
Address for correspondence: Asst. Prof. Yogesh Saxena, Associate Professor, Department of Physiology, Himalayan Institute of Medical Sciences, SRHU, Dehradun 248 140, Uttarakhand, India. E-mail: drysaxena@rediffmail.com
This is an open access article distributed under the terms of the Creative Commons Attribution-NonCommercial-ShareAlike 3.0 License, which allows others to remix, tweak, and build upon the work non-commercially, as long as the author is credited and the new creations are licensed under the identical terms.
This article was originally published by Medknow Publications & Media Pvt Ltd and was migrated to Scientific Scholar after the change of Publisher.
Abstract
Background:
Central nervous system (CNS) involvement is insidious and may occur early in subclinical hypothyroid (SCH) state which can be picked up by electrophysiological study. This study aims to record visual evoked potential (VEP), event-related latency and cognitive functions, and find their association with the levels of serum thyroid-stimulating hormone (TSH) in patients with SCH.
Materials and Methods:
In this cross-sectional study, 36 adult SCH patients and an equal number of age- and sex-matched euthyroid controls were included. Pattern reversal VEP, visual reaction time (RT), digit spanning test, and AB clock test (ABCT) were done in both SCH cases and euthyroid controls. The observed values were analyzed for comparison of mean values between the groups and correlation of recorded variables with the levels of serum TSH.
Results:
SCH cases showed a higher P100 (VEP) latency in both the right (103.2 ± 12.3 vs. 102.7 ± 6.8 ms) and left eye (101.1 ± 9.1 vs. 96.2 ± 10.7 ms) as compared to controls, but the difference was statistically insignificant. A significant delay in RT was observed on visible spectra of light in SCH cases (P < 0.001). Digit spanning score (forward and backward) in SCH cases was significantly lower than controls (P < 0.001), and a lower standardized score (<124 or <95th percentile) was significantly associated with SCH state (P = 0.027). No significant difference was observed in visuospatial domain by ABCT between both the groups although the median score was lower in SCH cases. Only digit spanning score showed a significant negative correlation with TSH levels (r = −0.4; P = 0.001).
Conclusion:
Decline in working memory and RT to visual stimuli is an evidence of the involvement of CNS in SCH. Prolonged latency in VEP may depend on the duration of SCH.
Keywords
Cognitive function
subclinical hypothyroidism
thyroid-stimulating hormone
visual evoked potential
INTRODUCTION
Hypothyroidism is a multiorgan endocrine disorder which results in metabolic dysfunction involving brain, nerves, heart, and muscular systems.[12] Neurological abnormalities of the central and peripheral nervous system occur in conjunction with systemic abnormalities in hypothyroid[34] and are present in 30%–80% of cases[5] but are noted only incidentally. Moreover, some signs and symptoms of neurological manifestations such as somnolence, impaired memory and concentration, neuropathy, and depression may sometimes contribute significantly to disability.[67]
Peripheral nervous system abnormalities have been studied in hypothyroid patients by variety of techniques.[89] On the contrary, quantification of central nervous system (CNS) dysfunction has not been investigated much. Hypothyroidism has been reported to affect both electroencephalogram[10] and visual evoked potential (VEP) in response to flash stimulation, causing particularly the delay in conduction.[1112] Recent studies have even established the reversibility of delay in VEP sensory conduction in hypothyroid patients following the appropriate thyroxin replacement[1314] using electrodiagnostic techniques.[4]
Clinical hypothyroidism in middle age is also associated with decrease in cognitive functions, especially memory. Cognitive tests of patients with moderate to severe hypothyroidism indicate difficulties in performing calculations, recent memory loss, reduced attention span, and slow reaction time (RT).[1516] These are not attributable to attention deficit but to specific retrieval deficits[17] after excluding interfering factors related to the perception of disease.[18] Hypothyroid patients showed prolongation of latencies only in the early event-related potential components, with speeding of sensory and cognitive processing after treatment.[19]
The insidious and steady history of hypothyroidism in adults indicates that it is a process which starts early but probably goes unnoticed. Subclinical hypothyroid (SCH) is asymptomatic and frequently a laboratory diagnosis stage with normal levels of free triiodothyronine (FT3) and T4 but a mildly increased serum thyroid-stimulating hormone (TSH) levels (≥4.25 µIu/mL). It has been useful to identify individuals at high risk of development to overt hypothyroid. Few recent studies have reported potential risk of development of neuropsychiatric dysfunction especially memory[920] and event-related latency[19] in SCH, but the association with serum TSH remains largely unexplored. As electrophysiological study could possibly be useful in documenting the subtle abnormalities, this study was planned to assess the VEP and cognitive function of memory and RT in SCH subjects and find their association, if any, with the levels of serum TSH.
MATERIALS AND METHODS
A cross-sectional analytical study on adult SCH subjects was conducted in the Department of Physiology, Himalayan Institute of Medical Sciences (HIMS), SRHU, Dehradun, after approval from the Institutional Ethical Committee. Written informed consent was taken from the subjects for inclusion in the study.
Sample size and sampling method
The study volunteers were selected by method of probability sampling. Using the mean values of P100 latency (VEP) in hypothyroid cases (96.9 ± 6.0 mm) and euthyroid controls (90.03 ± 2.7 mm),[21] sample size of 36 was calculated by formula for mean difference, two-sided test with alpha error of 0.05 and power of 90%. An equal number of age- and sex-matched euthyroid controls were also recruited (n = 36).
Selection of subjects
Equally literate, clinically healthy adults diagnosed with SCH in the age group of 20–40 years were recruited from the medical OPD of HIMS Hospital after they fulfilled the inclusion criteria: Diagnosed as SCH as per levels of serum TSH >5 µIU/ml up to 15 µIU/ml[22] and normal values of serum FT3 and free thyroxine (FT4). Diagnosis was based on the confirmatory biochemical parameters (serum FT3, FT4, and TSH) in our hospital. The normal ranges of serum FT3, FT4, and TSH were 2.0–4.2 pg/ml; 0.6–1.7 ng/dl, and 0.34–4.24 µIU/ml. Equal numbers of clinically healthy age- and sex-matched euthyroid controls were recruited from the attendants of patients and residents in and around SRHU campus. Common exclusion criteria were used to exclude the participants having diabetes mellitus, hypertension, bipolar disorder, tuberculosis, anemia, recent delivery (≤9 months), pregnancy, obesity (body mass index [BMI] ≥30 kg/m2), multiple endocrine syndromes, neuromuscular disorder, severe myopia, cataract, glaucoma, and maculopathy by detailed clinical history and systemic examinations including eye. Following investigations were done: Fasting blood sugar, X-ray chest, electrocardiogram, and hemoglobin estimation. Other investigations were done as required as per the specific exclusion criteria. Patients with any clinical symptoms or signs referable to CNS dysfunction, smokers, alcoholics and those taking drugs affecting thyroid status (lithium, nonsteroidal anti-inflammatory drugs) or drugs acting on CNS were also excluded from the study.
Study tools
Structured case reporting form was used to collect relevant clinical history, demographic, and anthropometric data. Neuropack Octopus (Nihon Kohden Japan) was used to measure the latency, and amplitude of VEP wave form. AB clock test (ABCT) and digit spanning test were used for cognitive impairment assessment. Multiple Choice Apparatus Model No. Medicraft 653 MP was used for visual RT (VRT). Bio Impedance Machine was used to measure BMI, % body fat, and visceral fat. Fixed metered scale and weighing machine (Krups) were used to measure height and weight. Noninvasive techniques were used to ensure that no discomfort was caused to the volunteers.
Study protocol
Volunteers were asked to report to the Physiology Department in morning hours on all working days. A standard case reporting form was administered by the investigator at the point of entry to collect information on demographic and anthropometric characteristics such as height, weight, BMI, personal medical history of past and present illness, and family history with detailed history of chronic medication, visual history related to diseases affecting vision, addiction, and smoking. Volunteers were familiarized with the procedures to be carried out.
Assessment of visual evoked potentials by Neuropack Octopus
Experimental procedure
Disc skin electrodes on scalp were used for recording VEPs. The scalp electrodes were placed relative to bony landmarks according to OCTOPUS system. A reversal flash pattern of checks with rate of 2 per se cond was used and values were recorded by computer-based program.[23] The subjects were properly instructed and motivated to provide full cooperation. They were instructed to have their scalp oil free and to avoid beverages and strenuous exercise on the day of recording. They were seated comfortably in an air conditioned and sound proof dark, quiet room and instructed to fixate their one eye (other being closed with a patch) on the central red spot of the TV monitor, kept at one-meter distance. Before commencement of the test, the subject was preadapted to the luminance of the blank screen for 5 min. The function of central visual pathway was evaluated by pattern VEP (PVEP). The recordings were done in the forenoon from 10 am to 12 noon after a light breakfast in the sitting posture. The subject was grounded properly. The skin of the scalp, the electrode disc, and electrical impedance were checked for proper functioning. All the subjects underwent a thorough eye examination and refraction was corrected to 6/6. The subjects were allowed to wear the usual spectacles (if any) during the test. The black and white checks (15 mm × l5 mm size) subtending an angle of 32 min of an arc, were generated on the monitor through electronic generator of the Evoked potential recorder. The electrical activity had low cut of 2 Hz and high cut of 0.3 kHz filters. The sweep speed was 50 ms/division. Then, monoocular stimulation was chosen with flash checkerboard. The evoked responses were recorded and averaged after giving a trial of 256 checker board stimuli. Two such trials for each eye were given and responses superimposed to demonstrate replicability. The Visual Evoked Potential - Positive (VEP-P) complexes were analyzed in terms of absolute peak latencies and PI amplitude. Latencies were used to analyze the differences between cases and controls.
Assessment of cognitive test by AB clock test and digit spanning test
Experimental procedure
A cognitive function involving visuospatial skills was tested by ABCT,[24] and working memory was tested by digit spanning test.[25]
ABCT – investigator by the gesture with his/her finger asked the subject to draw with pencil, a circle of modest size on the given blank sheet of paper. When the circle was drawn, subject was asked to put in the numbers as in the face of a clock and set the hands of clock to show “ten past eleven.” It was prompted at each stage. “Put in the numbers.put the time as ten past eleven.” Scoring template was placed over the completed clock with the template's “12 o’clock” line placed over the subject's 12. The template was adjusted to maximize the score for the numbers and hands. Recording of scores was done as per the instructions of the ABCT questionnaire.
The digit memory test was done to investigate verbal memory difficulties. Subject was asked to listen to some numbers at the rate of one number per second carefully and later asked to repeat the digits in monotone without any variation in pitch of voice. The Same procedure was repeated with the instruction to repeat the digits backward. The individual score was the total number of items correctly repeated forward and backward. Both the forward and backward scores were added together and compared with standard chart for final score. Ninety-five percentile standard score value of 124 was set as cutoff for memory difficulties.
Assessment of visual reaction time by multiple choice apparatus
Experimental procedure
RT was recorded by visual multiple choice RT apparatus (Model No. Medicraft 653 MP) with a sensitive clock (measures time up to 1/10th ms; Accuracy: + one digit). Apparatus is equipped with four light stimuli (red, blue, green, and yellow). After familiarizing oneself with the procedure, apparatus and demonstration, three practice trials were given to every volunteer before final recording. The readings of VRT were recorded in a quiet adequately illuminated room with subject sitting comfortably in the chair at a constant distance from instrument. Random visual signals were presented, and subjects were instructed to respond to the flashing of the light immediately by pressing switch-off knob on the panel. Three readings were recorded, and minimum RT was taken as the final RT for that sensory modality of that subject.[26]
Data management and statistical analysis
SPSS (IBM SPSS Statistics for Windows, Version 19.0. Armonk, NY: IBM Corp) was used to analyze the collected data. Mean and proportions were used to represent the demographic and other measured variables. Quantitative data were represented as mean and SD and qualitative data as proportions and percentages. Differences in means of quantitative variables (e.g., VEP latency, amplitude, RT) in the groups were tested by unpaired t-test and qualitative variables (memory scores) by Mann–Whitney U-test. Correlation of the serum TSH with the continuous variables, for example, VEP latency and RT were assessed by Pearson product moment correlation coefficient and with the qualitative variables such as memory scores by Spearman correlation. P < 0.05 was taken as statistically significant.
RESULTS
Thirty-six adult cases (20–40 years of age) diagnosed as having SCH were studied for Evoked potentials and cognitive functions. An equal number of age-matched controls were taken for comparison.
The cases and controls had comparable height, weight, and obesity markers - BMI, visceral fat, and % body fat. The number and proportion of females were considerably more than males, i.e., 31 (86.1%). A significant difference was seen between serum TSH levels of SCH cases and euthyroid controls (P ≤ 0.001). No significant differences regarding FT3 and FT4 levels were observed between SCH cases and controls [Table 1].
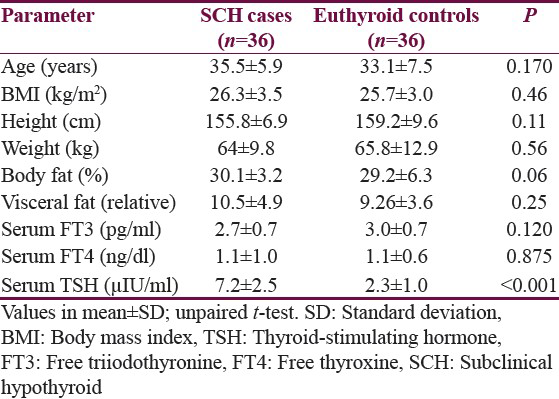
CNS affection was observed by comparing the P100 and N135 latency of VEPs for both cases and controls. No significant differences were seen regarding P100 latencies in both the eyes among cases and controls (P > 0.05 each) although the duration of latency in SCH cases was more than the euthyroid controls. N135 latency period was higher in SCH cases as compared to controls in the right eye (P = 0.035) but not in the left eye (P = 0.169) [Table 2].

Significantly, lower standard digit spanning score was observed in SCH cases as compared to euthyroid controls (P < 0.001). Normal (≥124 standard score) value of digit spanning test was observed among 8 controls as compared to only 1 case. Below normal (<124 standard score) value was observed in higher number of cases than controls 35 out of 36 as compared to control. SCH cases were significantly associated with below normal standard digit spanning score (<124) in our study (P = 0.027) [Table 3]. No significant difference was observed in visuospatial domain by the ABCT between euthyroid control and SCH cases.
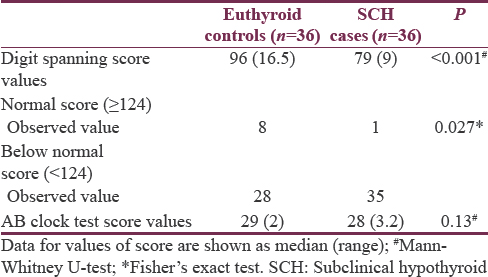
RT to visual stimuli for all the primary colors of the visible spectra showed a slower response time in SCH cases in comparison to controls, and the differences were statistically highly significant (P < 0.01 each) [Table 4].
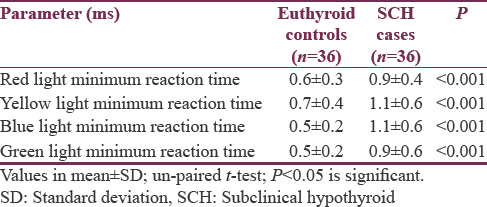
Serum TSH had significant negative correlation with digit spanning score (r = −0.40, P = 0.001). Although the serum TSH levels had negative correlation with latency in generation of N75 (r = −0.10, P = 0.57), P100 (r = −0.053, P = 0.78), and N135, (r = −0.11, P = 0.43) potential waves, the correlation was nonsignificant.
DISCUSSION
Neurological alterations in hypothyroidism have shown that CNS is more vulnerable to the effects of hypothyroidism than the peripheral nervous system. Therefore, electrophysiological neurological studies can be performed in hypothyroid subjects early in the course of thyroid deficiency to detect nervous system involvement. Evoked potentials such as VEPs provide a reliable and objective measure of function in related sensory system and tracts.[9]
The normal response to pattern stimulation is a triphasic response with a prominent positive wave (P100) with a peak latency of 84–105 (mean: 96 ± 4) ms which is suggestive of CNS activity. The P100 waveform is generated in the striate and peristriate occipital cortex due to the activation of the primary visual cortex and also due to the discharge of the thalamocortical fibers. The latency depends on salutatory conduction due to myelination, whereas the amplitude of wave primarily on number of functioning axons in the nerve. Slow conduction or increased latency usually implies defects in myelination and loss of amplitude due to axonal dysfunction. The P100 is a prominent peak that shows relatively little variation between the subjects, minimal within inter-ocular difference, and minimal variation with repeated measurements over time. Therefore, the present study focused more on the P100 latency values among the study groups, and its correlation with the levels of serum TSH.
The mean N75 values in controls and cases for both the eyes were not significantly different. The mean P100 latency for the monocular vision from both the right and left eye was marginally higher in cases as compared to controls but was statistically nonsignificant. Nazliel et al. in their study on pattern-shift VEP in 48 newly diagnosed hypothyroid patients also observed nonsignificant difference between overt hypothyroid and SCH, but a marginally higher latency of P100 was observed in hypothyroid cases (mean: 97 ± 6 ms) as compared to controls (96 ± 4 ms).[9] Significantly prolonged latency in P100 was observed in several studies in hypothyroid cases in both the eyes[21] which reduced following treatment with triiodothyronine.[27] Also not all cases of hypothyroidism present with prolonged latency in P100 as observed by Khedr et al.[4] in 52% of age- and sex-matched hypothyroid cases. The increase in P100 latency may vary with number of checks in the PVEP in hypothyroid cases as observed by Osterweil et al., who found increased P100 latency in only 20 checks of PVEP and not in 50 checks[28] and attributed it to central retinal dysfunction. The above studies have observed changes in latency of P100 (VEP) in long-standing hypothyroidism or untreated patients with obvious neurological involvement. It may be possible that SCH duration may not be long enough in our study and changes in myelination may be very subtle to affect VEP latency.
RT is the time interval between onset of stimulus and occurrence of response. It is an important parameter representing the integration of sensory, motor, and coordination system of the body.[29] In our study, there was a significant increase in VRT for all the spectra of light in SCH patients as compared to the control group. Our findings in SCH cases are similar to the findings of del Ser Quijano et al.[30] Shah and Nahar in their study found a significant increase in both audio and VRT in hypo as well as hyperthyroid patients.[29] VRT was significantly increased in hypothyroid patients even in middle age (40–50 years) as reported by Vedavathi et al. (P < 0.01).[27] The long RT in hypothyroid and SCH patients can be explained because of generalized decrease in metabolic rates affecting the sensory receptors, neural pathways, and skeletal muscles.[29] Measurement of VRT is a simple procedure and can act as a sensitive indicator of thyroid dysfunction in SCH, in the absence of any other clinical pathology.
Cognitive assessment using digit spanning test for working memory in SCH cases observed a median score of 79 (interquartile range [IQR] = 9), which was lower than the median score in euthyroid controls (median = 96, IQR = 16.5). Complementing our study, del Ser Quijano et al. also found that SCH cases performed significantly worse in the verbal, visual memory, and global cognitive performance but improved on thyroxin replacements.[30] Similar findings were observed by Sunita et al. across the young and elderly SCH otherwise normal population in both forward and backward digit test[31] and by Martino and Strejilevich in bipolar disorder subjects where the SCH showed a poorer performance with cognitive function of verbal memory and attention.[32] A recent longitudinal study in the adults has observed that a decline in the digit spanning backward test (reflecting working memory) and clock–command test of visuospatial and construction was linked to the above range of TSH levels in SCH subjects.[33] SCH was associated with lower standardized score 120 (<95th percentile score of 124) of digit spanning test in our study (P = 0.02) but failed to find any association with visuospatial ABCT. We did not find any significant relationship of cognitive variables with serum TSH levels. It can be said that working memory is slightly less in cases as compared to controls, which could be because of slow processing due to modulation of calorigenesis and oxygen consumption[34] leading to decrease in metabolic rates. It may also be explained by slow neural conduction and transduction at the sensory receptors, due to myelination and decreased voltage-gated sodium current density resulting in decreased slope of action potential[35] as observed in overt hypothyroid cases.
Thyroid deficient subjects have decline in working memory and RT to stimuli which is an evidence of involvement of CNS much earlier in stage and which may increase with the duration of elevated TSH. We have tried to rule out the subtle neurological risk factors in SCH subjects along with defect in visual equity before the VEP recording, yet we could not establish long latency in P100 in them since the duration of symptoms before diagnosis was not considered. Periodic evaluation of neurological tests may help in earlier management of symptoms before the person becomes clinically hypothyroid. A follow-up study of VEP on SCH subjects is needed to provide evidence for the effect of high TSH on sensory conduction in CNS.
CONCLUSION
Decline in working memory and RT to visual stimuli is an evidence of the involvement of CNS in SCH. Prolonged latency in VEP may depend on the duration of SCH.
Financial support and sponsorship
The authors acknowledge SRHU for providing the financial support and Logistics for the study.
Conflicts of interest
There are no conflicts of interest.
Acknowledgment
The authors acknowledge Himalaya Institute of Medical Sciences for providing the financial support and Logistics for the study.
REFERENCES
- Neurological Manifestations of Thyroid and Parathyroid Disorders. New York (NY): WB Saunders; 2010. p. :609-12.
- Thyroid disease and the nervous system. In: Neurology and General Medicine (Livingstone second edition). 1995. p. :333-47.
- [Google Scholar]
- Peripheral and central nervous system alterations in hypothyroidism: Electrophysiological findings. Neuropsychobiology. 2000;41:88-94.
- [Google Scholar]
- The neuromuscular system and brain in hypothyroidism. In: Braverman LE, Utiger RD, eds. The Thyroid. A Fundamental and Clinical Text. Philadelphia: Lippincott Williams & Wilkins; 2000. p. :806.
- [Google Scholar]
- The thyroid: Physiology, thyrotoxicosis, hypothyroidism, and the painful thyroid. In: Felig P, Frohman LA, eds. Endocrinology & Metabolism. USA: McGraw-Hill Companies; 2001. p. :261.
- [Google Scholar]
- Iodothyronine levels in the human developing brain: Major regulatory roles of iodothyronine deiodinases in different areas. J Clin Endocrinol Metab. 2004;89:3117-28.
- [Google Scholar]
- Pattern visual evoked potential (PVEP) evaluation in hypothyroidism. J Endocrinol Invest. 2002;25:955-8.
- [Google Scholar]
- Reversible alterations of peripheral nerve function in thyroid dysfunction (author's transl) EEG EMG Z Elektroenzephalogr Elektromyogr Verwandte Geb. 1979;10:101-5.
- [Google Scholar]
- The changes of central and peripheral nerve conduction and the effect of thyroxine replacement in thyroidectomized rats. Gaoxiong Yi Xue Ke Xue Za Zhi. 1995;11:589-98.
- [Google Scholar]
- Visual evoked potentials in hypothyroid and hyperthyroid patients before and after achievement of euthyroidism. J Endocrinol Invest. 1992;15:749-53.
- [Google Scholar]
- A longitudinal study of central and peripheral nerve conduction in hypothyroid rats. J Neurol Sci. 1987;89:139-45.
- [Google Scholar]
- Visual, brainstem auditory and somatosensory evoked potential abnormalities in thyroid disease. Thyroidology. 1989;1:137-42.
- [Google Scholar]
- Hypothyroidism and cognition: Preliminary evidence for a specific defect in memory. Thyroid. 2001;11:1177-85.
- [Google Scholar]
- Verbal memory retrieval deficits associated with untreated hypothyroidism. J Neuropsychiatry Clin Neurosci. 2007;19:132-6.
- [Google Scholar]
- Psychopathological and cognitive features in subclinical hypothyroidism. Prog Neuropsychopharmacol Biol Psychiatry. 1997;21:925-35.
- [Google Scholar]
- Cognitive status in hypothyroid female patients: Event-related evoked potential study. Neuroendocrinology. 2008;88:59-66.
- [Google Scholar]
- Neuromuscular findings in thyroid dysfunction: A prospective clinical and electro diagnostic study. J Neurol Neurosurg Psychiatry. 2000;68:750-5.
- [Google Scholar]
- Pattern reversal visual evoked potentials in hypothyroid females. Natl J Basic Med Sci. 2012;1:365-8.
- [Google Scholar]
- Subclinical thyroid disease: Scientific review and guidelines for diagnosis and management. JAMA. 2004;291:228-38.
- [Google Scholar]
- ISCEV standard for clinical visual evoked potentials: (2016 update) Doc Ophthalmol. 2016;133:1-9.
- [Google Scholar]
- Reliability and validity of the AB clock test (ABCT): An objective approach to scoring the clock drawing test. Clin Res. 2006;3:16-21.
- [Google Scholar]
- A preliminary investigation of the running digit span as a test of working memory. Behav Neurol. 2008;20:17-25.
- [Google Scholar]
- Comparative study of simple and choice visual reaction time on medical students of Bhavnagar region. IRJP. 2012;3:334-5.
- [Google Scholar]
- Reaction time study as a tool to identify central nervous system affect due to hypothyroidism. IJHSR. 2013;3:29-32.
- [Google Scholar]
- Cognitive function in non-demented older adults with hypothyroidism. J Am Geriatr Soc. 1992;40:325-35.
- [Google Scholar]
- Reaction time in hypothyroid and hyperthyroid patients before and after drug treatment. IOSR J Pharm. 2012;2:218-21.
- [Google Scholar]
- Cognitive function in young female with subclinical hypothyroidism. Int J Physiol. 2015;3:28-33.
- [Google Scholar]
- Subclinical hypothyroidism and neurocognitive functioning in bipolar disorder. J Psychiatr Res. 2015;61:166-7.
- [Google Scholar]
- Thyroid hormones are associated with longitudinal cognitive change in an urban adult population. Neurobiol Aging. 2015;36:3056-66.
- [Google Scholar]
- Thyroid hormone effects on sensory perception, mental speed, neuronal excitability and ion channel regulation. 2012. Thyroid Hormone. (ed first). Croatia: In Tech; :86. Available from: THYROID HORMONElibrary.umac.mo/ebooks/b28356354.pdf
- [Google Scholar]