Translate this page into:
Multi-contrast echo-planar imaging sequence (Echo-planar imaging mix) in clinical situations demanding faster MRI-brain scans
*Corresponding author: Chandrasekharan Kesavadas, Department of Imaging Sciences and Interventional Radiology, Sree Chitra Tirunal Institute for Medical Sciences and Technology, Thiruvananthapuram, Kerala, India. chandkesav@yahoo.com
-
Received: ,
Accepted: ,
How to cite this article: Kalaparti Sri Venkata Ganesh V, Kamepalli H, Sharma D, Thomas B, Kesavadas C. Multi-contrast echo-planar imaging sequence (Echo-planar imaging mix) in clinical situations demanding faster MRI-brain scans. J Neurosci Rural Pract. 2024;15:341-8. doi: 10.25259/JNRP_508_2023
Abstract
Objectives:
The excellent resolution offered by magnetic resonance imaging (MRI) has a trade-off in the form of scan duration. The purpose of the present study was to assess the clinical utility of echo-planar imaging mix (EPIMix), an echo-planar imaging-based MRI sequence for the brain with a short acquisition time.
Materials and Methods:
This was a retrospective observational study of 50 patients, who could benefit from faster MRI brain scans. The T1, T2, fluid attenuated inversion recovery, diffusion-weighted imaging (DWI), and T2*/susceptibility-weighted imaging sequences were acquired, conventionally and with EPIMix. Conventional and EPIMix images were assessed by two radiologists for overall quality, motion, and susceptibility artifacts and scored on a Likert scale. The scores given for conventional and EPIMix images were compared. The diagnostic performance of EPIMix was also assessed by the ability to detect clinically relevant findings.
Results:
The acquisition time for conventional MRI was 11 min and 45 s and for EPIMix 1 min and 15 s. All EPIMix images were sufficient for diagnostic use. On assessment of the diagnostic performance, it was excellent for ischemic and hemorrhagic strokes. Smaller lesions, lesions adjacent to bone, and post-operative tumors were difficult to identify. Moderate to perfect agreement (Kappa values 0.41–1) was seen between radiologists for all categories except skull base, calvarial, and orbital lesions. Image quality, artifact assessment showed excellent interobserver agreement (>90%) for the scores. All EPIMix images showed reduced motion artifacts. The EPIMix-DWI was comparable to conventional-DWI in terms of quality and artifacts. The remaining sequences showed reduced quality and increased susceptibility.
Conclusion:
The EPIMix has a significantly reduced acquisition time than conventional MRI and could be used instead of conventional MRI in situations demanding faster scans such as suspected acute ischemic or hemorrhagic stroke. In other clinical scenarios, it could help tailor the MRI examination for each patient.
Keywords
Magnetic resonance imaging
Neuroimaging
Stroke
INTRODUCTION
The excellent resolution offered by magnetic resonance imaging (MRI) has a trade-off in the form of scan duration. This has become more apparent with some of the novel sequences that either require additional gradients or have a low tolerance for motion.[1] There are two main problems with these long acquisition times. The first is establishing the utility of MRI in clinical scenarios demanding rapid scans. The second is the movement of uncooperative patients within the gantry leading to motion artifacts. To reduce scan time, investigators have put forth various solutions.[2] This study focuses on a multi-contrast echo planar imaging-based sequence, echo-planar imaging mix (EPIMix) with a significantly reduced scan time.[3]
To reduce the scan time and address motion during the scan, the previous studies have modified the acquisition parameters and techniques.[4,5] The EPIMix has been shown to further shorten the scan duration in two volunteers.[3] Only a few studies have been conducted to evaluate the clinical utility of EPIMix.[6,7] In our institutional experience, where pediatric and other uncooperative patients form a major proportion of the MRI examinations, EPIMix could be more useful.
We hypothesize that EPIMix could have comparable image quality to conventional sequences. The present study aims to assess the clinical utility of EPIMix by comparing it to conventional sequences in various clinical scenarios.
MATERIALS AND METHODS
Study plan
This was a retrospective observational study of 50 consecutive patients. The inclusion criteria were patients, who were uncooperative for MRI brain scans including some claustrophobic, post-operative patients, those with altered sensorium, with painful conditions preventing them from lying still in the MRI gantry, and with suspected stroke/transient ischemic attack (TIA). As per institutional protocol, EPIMix was done in addition to the conventional MRI sequences in these patients when it was anticipated that the conventional images would be degraded by motion artifacts. The exclusion criteria were any contraindications for MRI or the ability to undergo the full MRI protocol normally. Institutional ethics committee approval was obtained before starting the study. Consent was waived, as the study was retrospective.
Both the conventional and EPIMix MRI scans were performed on a 3 Tesla Discovery 750w MRI machine (GE Healthcare, Milwaukee, Wisconsin, USA). None of the patients was sedated or anesthetized before the MRI. The total acquisition time of a conventional MRI was 11 min and 45 s. For EPIMix, it was 1 min and 15 s. The EPIMix was acquired randomly between the conventional sequences and the point of acquisition differed between patients. In cases with suspected intracranial space-occupying lesions (ICSOLs), EPIMix was acquired after administration of contrast. The acquisition protocols are detailed in Supplementary Tables 1 and 2.
Evaluation of the diagnostic performance
Subjects and their images were separated into pre-defined categories based on clinically relevant imaging findings on conventional images by one of the authors not involved in reading the EPIMix images (DPS). Imaging findings due to chronic pathologies such as small vessel ischemic changes, chronic infarcts, and microbleeds that could not explain the symptoms at clinical presentation were excluded from this categorization for ease of analysis. The EPIMix images were read by two radiologists (VKSVG and HKK), who were blinded to the patient details, the clinical history, and the types of images being presented to reduce bias. Blinding was also ensured by presenting the images to the readers randomly rather than consecutively. The number of cases in which the relevant findings were picked up in each of the categories was recorded.
Analysis of the image quality, artifacts
Conventional and EPIMix images were also assessed for quality by the two radiologists (VKSVG and HKK) after randomization, being blinded to the type of sequence and the clinical history. For both the conventional and EPIMix acquisitions, the sequences T1, T2, fluid-attenuated inversion recovery (FLAIR), diffusion-weighted imaging (DWI), and T2* were compared based on overall quality and two artifact parameters. The overall quality had a possible score of 1–4 on the Likert scale. The scoring system was based on a previous study.[7] A poor image quality, not acceptable for diagnostic use was assigned a score of 1. A score of 2 was for images of sufficient quality for diagnostic use, but with minor issues. A score of 3 was for good-quality images, and a score of 4 was for excellent-quality images, acceptable for diagnostic use. The two artifact parameters that were analyzed were motion and susceptibility. Motion artifacts were chosen to assess the capability of EPIMix to reduce them. Susceptibility artifacts were selected, as echo-planar imaging-based sequences are more prone to them. These were the artifacts that hampered diagnostic accuracy in most cases in the clinical setting. Artifacts were again scored from 1 to 4 on the Likert scale. A score of 1 was for severe artifacts with the images not acceptable for diagnostic use. A score of 2 was for moderate artifacts with the images being acceptable for diagnostic use. A score of 3 was for mild artifacts, and a score of 4 was for images with no artifacts, both types of images being acceptable for diagnostic use.
Statistical analysis
For the analysis of diagnostic performance, the percentage detection rate, agreement percentage, and kappa value were calculated for each category.
For the analysis of image quality and artifacts, percentage agreement and a mean score were calculated for the image quality and artifact parameters in each sequence from scores given by the two radiologists. Each of these mean scores for each assessment parameter in each EPIMix sequence was compared with its counterpart parameter in the corresponding conventional sequence. Wilcoxon signed-rank test was used for this comparison. Categorical paired variables were compared with the McNemar test. P < 0.05 was taken as statistically significant.
RESULTS
Demographic characteristics
A total of 50 patients were included in the study, 27 of them being males and 23 females. The mean age of the study population was 42.14 ± 18.82 years (Mean ± Standard deviation).
The major indications for MR examinations were suspected acute stroke or TIA (14/50, 28%), post-operative scan after resection of an ICSOL (11/50, 22%), seizures (8/50, 16%), evaluation of a suspected ICSOL (6/50, 12%), evaluation of an intracranial vascular malformation (5/50, 10%), headache (3/50, 6%), loss of consciousness (2/50, 4%), and visual disturbances (1/50, 2%).
Evaluation of the diagnostic performance
The categories defined for the evaluation of the diagnostic performance of EPIMix are summarized in Table 1.
Category | Lesions detected by Radiologist 1 (%) | Lesions detected by Radiologist 2 (%) | Percentage agreement | Kappa value |
---|---|---|---|---|
Normal (n=15) | 15/15 (100) | 15/15 (100) | 100 | N/A* |
Ischemic stroke (n=6) | 6/6 (100) | 6/6 (100) | 100 | N/A* |
Hemorrhage (n=2) | 2/2 (100) | 2/2 (100) | 100 | N/A* |
Vascular malformation (n=4) | 2/4 (50) | 2/4 (50) | 100 | 1† |
Epileptogenic lesions (MTS, FCD, tubers) (n=7) | 3/7 (42.8) | 2/7 (28.5) | 87.5 | 0.695† |
Intracranial tumors (pre-and post-operative) (n=12) | 10/12 (83.3) | 10/12 (83.3) | 83.33 | 0.4† |
Skull base, calvarial and orbital lesions (n=4) | 2/4 (50) | 2/4 (50) | 50 | 0† |
Overall (n=50) | 40/50 (80) | 39/50 (78) | 88 | 0.625† |
MTS: Mesial temporal sclerosis, FCD: Focal cortical dysplasia, EPIMix: Echo-planar imaging mix. *Not calculable †Kappa values 0: No agreement, 0.01-0.2: Slight agreement, 0.21-0.4: Fair agreement, 0.41-0.6: Moderate agreement, 0.61-0.8: Substantial agreement, 0.81-1: Almost perfect or perfect agreement, , N/A*: Not applicable
Normal MR images (n = 15), ischemic strokes (n = 6), and intraparenchymal hemorrhages (n = 2) were identified in all patients with good percentage agreement among the radiologists.
The vascular malformations (n = 4) were identified by the presence of abnormal flow voids and included one dural arteriovenous fistula, one vein of Galen malformation, and two developmental venous anomalies. The dural arteriovenous fistula and the vein of Galen malformation were identified, but the developmental venous anomalies (both just under 15 mm in size) could not be identified by either radiologist.
Among the epileptogenic lesions (n = 7), there were two cases with mesial temporal sclerosis, four cases with focal cortical dysplasia (all below 10 mm in size), and one case with cortical tubers (more than 30 mm in size). Both radiologists could not identify the cortical dysplasias. Both could, however, identify cortical tubers and diagnose mesial temporal sclerosis in one case. Only one radiologist identified the sclerotic hippocampus in the other case while the second radiologist reported it as normal.
Tumors or tumor-like lesions (all >20 mm in size) were identified by the presence of a focal lesion with mass effect and were divided into two categories, intracranial (n = 12) and skull base, calvarial, and orbital tumors (n = 4) based on the observation that detection of lesions with the bone surrounding them was difficult. These categories included both pre-and post-operative lesions on follow-up. Relevant finding in the pre-operative lesions was defined as the identification of the presence and the site of the lesion while in post-operative lesions; it was the possibility of a residual or recurrent lesion, based on a focal mass lesion with or without enhancement adjacent to the resection cavity.
All the intracranial tumors were diffuse infiltrative gliomas or suspected gliomas. Four were pre-operative lesions, two in the left temporal lobe, one in the right temporal lobe, and one in the left frontal lobe. Two were post-operative cases with possible residual or recurrent lesions and six post-operative cases without obvious residual or recurrent lesions. All pre-operative lesions could be identified by both radiologists. One post-operative recurrence was missed by the second radiologist. The first radiologist found it difficult to ascertain the absence of a residual or recurrent lesion after surgery in two patients while the second radiologist faced the same difficulty in one patient.
Among the skull base, calvarial, and orbital lesions, there were three pre-operative lesions, two frontal calvarial lesions, and one orbital lesion. The first frontal calvarial lesion was identified only by the first radiologist, while the second lesion was identified only by the second radiologist. The orbital lesion was detected by both. There was one post-operative clival chordoma with a residual lesion that was missed by both radiologists.
Overall, the first reader was able to identify 80%, and the second reader could identify 78% of the clinically relevant imaging features in EPIMix images. The percentage agreement between the radiologists was good, and the calculation of the kappa value showed substantial agreement.
Quantitative assessment of the image quality and artifacts
There was good percentage agreement (≥90%) for the scores given by both radiologists to the overall quality, and artifact parameters in both types of sequences [Supplementary Tables 3 and 4].
The mean scores given by the two radiologists for the overall quality, motion, and susceptibility artifacts for EPIMix images and the corresponding conventional images are shown in Table 2. Upon analysis, a statistically significant difference between the mean scores of EPIMix and conventional images was observed for the following criteria- overall quality, motion, and susceptibility artifacts in T1, T2, FLAIR, and T2* and motion artifacts in DWI.
T1 | |||||||
---|---|---|---|---|---|---|---|
Parameter | EPIMix mean score [Mean(±SD)] | 95% confidence interval for mean | Conventional mean score [Mean(±SD)] | 95% confidence interval for mean | P-value* | ||
Lower bound | Upper bound | Lower bound | Upper bound | ||||
Overall quality | 2.89 (±0.25) | 2.81 | 2.96 | 3.95 (±0.15) | 3.9 | 3.99 | <0.001† |
Motion artifacts | 3.93 (±0.27) | 3.84 | 3.99 | 3.83 (±0.33) | 3.73 | 3.92 | 0.006† |
Susceptibility artifacts | 2.15 (±0.32) | 2.05 | 2.24 | 3.99 (±0.07) | 3.97 | 4 | <0.001† |
T2 | |||||||
Overall quality | 2.08 (±0.25) | 2 | 2.15 | 3.97 (±0.15) | 3.92 | 4 | <0.001† |
Motion artifacts | 3.96 (±0.17) | 3.85 | 3.99 | 3.88 (±0.29) | 3.79 | 3.96 | <0.001† |
Susceptibility artifacts | 2.54 (±0.44) | 2.41 | 2.66 | 3.97 (±0.12) | 3.93 | 4 | <0.001† |
FLAIR | |||||||
Overall quality | 2.85 (±0.32) | 2.76 | 2.94 | 3.97 (±0.15) | 3.92 | 4 | <0.001† |
Motion artifacts | 3.95 (±0.25) | 3.86 | 3.98 | 3.86 (±0.32) | 3.77 | 3.9 | <0.001† |
Susceptibility artifacts | 2.7(±0.44) | 2.57 | 2.82 | 3.97 (±0.15) | 3.92 | 4 | <0.001† |
DWI | |||||||
Overall quality | 3.93 (±0.24) | 3.86 | 4 | 3.9 (±0.28) | 3.82 | 3.98 | 0.7 |
Motion artifacts | 3.98 (±0.07) | 3.97 | 4 | 3.9 (±0.28) | 3.82 | 3.98 | <0.05† |
Susceptibility artifacts | 2.91 (±0.26) | 2.83 | 2.98 | 2.94 (±0.22) | 2.87 | 2.99 | 0.5 |
SWI/GRE | |||||||
Overall quality | 2.61 (±0.44) | 2.48 | 2.73 | 3.83 (±0.34) | 3.73 | 3.92 | <0.001† |
Motion artifacts | 3.98 (±0.14) | 3.94 | 4 | 3.56 (±0.44) | 3.44 | 3.7 | <0.003† |
Susceptibility artifacts | 2.54 (±0.47) | 2.4 | 2.67 | 2.95 (±0.2) | 2.9 | 3 | <0.001† |
N/A: Not Applicable, EPIMix: Echo-planar imaging mix, SD: Standard deviation, FLAIR: Fluid-attenuated inversion recovery, DWI: Diffusion weighted imaging, SWI: Susceptibility weighted imaging, GRE: Gradient echo. *Wilcoxon signed-rank test was used for analysis, †Statistically significant
Qualitative assessment of the image quality and artifacts
The mean values of the scores assessing overall quality and artifact parameters in the conventional and EPIMix images were again dichotomized. The minimum score that was needed for an image to be sufficient for diagnostic use was 2. In the quality assessment, none of the images was given a score of <2, and the dichotomization was done as <3 or ≥3, indicative of being acceptable or excellent for clinical use, respectively. For the artifact assessments, the minimum mean score given was again 2, and the dichotomization was done as <3 or ≥3, indicative of a severe degree or fewer artifacts, respectively. The dichotomized mean scores were compared with the McNemar test.
In comparison, the overall quality of conventional-T1 was higher than the EPIMix-T1 images, and the difference was statistically significant (P < 0.001). Motion artifacts were higher in conventional-T1 images than EPIMix-T1 images (P < 0.001), and susceptibility artifacts were higher in EPIMix-T1 images than conventional-T1 images (P = 0.032). The overall quality of EPIMix-T2 was lower than conventional-T2 (P = 0.01), motion artifacts were higher in conventional-T2 than EPIMix-T2 (P < 0.001), and susceptibility artifacts were higher in EPIMix images than conventional images (P = 0.038). The overall quality of EPIMix-FLAIR was lower than conventional-FLAIR (P < 0.001), motion artifacts were higher in conventional-FLAIR than EPIMix-FLAIR (P < 0.001), and susceptibility artifacts were higher in EPIMix-FLAIR than conventional-FLAIR (P = 0.001). The overall quality for EPIMix-T2* was lower than conventional-susceptibility-weighted imaging (SWI) (P = 0.004), and the undesirable susceptibility artifacts in EPIMix-T2* were higher than conventional-SWI (P = 0.013). When conventional-DWI and EPIMixDWI were compared, the quality and artifact parameters showed no significant difference.
DISCUSSION
The present study assessed the image quality, artifacts, and diagnostic performance of EPIMix images, compared to the conventional MR sequences. The total scan time for the EPIMix sequence was 1 min, and 15 s, significantly lower than the time for obtaining similarly weighted conventional images. We found that T1-FLAIR, T2, FLAIR, and T2* images generated by EPIMix were inferior in overall quality when compared to their conventional counterparts while still being sufficient for diagnostic use. When motion and susceptibility artifacts were assessed, EPIMix images of these sequences showed relatively increased susceptibility artifacts at the skull base and tissue-bone-air interfaces likely due to the increased vulnerability of EPI to magnetic field inhomogeneities. As for the motion artifacts, they were relatively lesser in degree in the EPIMix images [Figure 1]. The DWI images, however, did not show a significant difference in image quality.
![A 54-year-old male patient with hypophysitis, who presented with headache, diplopia. Conventional [top row, (a) T1W, (b) T2W, (c) FLAIR, (d) DWI, and (e) SWAN] and EPIMix (bottom row, (f) T1W, (g) T2W, (h) FLAIR, (i) DWI, and (j) T2*) MR axial images are shown. Overall, image quality of EPIMix images is lower than that of conventional images, except that of (i) EPIMix-DWI. The motion artifacts (white arrow in c) seen in the conventional images are lesser in the EPIMix images. The EPIMix images also suffer from increased incidence of susceptibility artifacts (white asterisks in f-j). T1W: T1-weighted, T2W: T2-weighted, FLAIR: Fluid-attenuated inversion recovery, DWI: Diffusion-weighted imaging, SWAN: Susceptibility-weighted angiography, EPIMix: Echo-planar imaging mix, MR: Magnetic resonance.](/content/150/2024/15/2/img/JNRP-15-341-g001.png)
- A 54-year-old male patient with hypophysitis, who presented with headache, diplopia. Conventional [top row, (a) T1W, (b) T2W, (c) FLAIR, (d) DWI, and (e) SWAN] and EPIMix (bottom row, (f) T1W, (g) T2W, (h) FLAIR, (i) DWI, and (j) T2*) MR axial images are shown. Overall, image quality of EPIMix images is lower than that of conventional images, except that of (i) EPIMix-DWI. The motion artifacts (white arrow in c) seen in the conventional images are lesser in the EPIMix images. The EPIMix images also suffer from increased incidence of susceptibility artifacts (white asterisks in f-j). T1W: T1-weighted, T2W: T2-weighted, FLAIR: Fluid-attenuated inversion recovery, DWI: Diffusion-weighted imaging, SWAN: Susceptibility-weighted angiography, EPIMix: Echo-planar imaging mix, MR: Magnetic resonance.
Increased acquisition time precludes MRI from being used in neurological emergencies such as trauma or non-traumatic hemorrhage, where computed tomography (CT) is still used. In acute ischemic stroke, where door-to-needle time is paramount and MRI is the imaging modality of choice in many centers, a prolonged scan time is undesirable. In uncooperative, critically ill, and claustrophobic patients, it is a challenge to complete the desired MR examination. Even in cases where the examinations are completed with great difficulty, they are riddled with motion artifacts and may prove to be non-diagnostic.[2,3]
An MR image is affected by motion due to the inconsistency of data in the various parts of the K-space.[8] This can be prevented using breath hold, sleep, sedation or general anesthesia.[9,10] Other techniques to reduce motion artifacts include parallel imaging, application of saturation bands on moving structures, using additional gradients, spiral, radial K-space filling or the PROPELLER technique.[11-14] Many of these measures have certain disadvantages.
Newer MRI techniques such as ultrafast MRI, echo-planar imaging (EPI)-based techniques or quantitative MRI that can significantly reduce the scan time have garnered attention.[4,15,16] The EPIMix is an EPI-based MRI sequence that can acquire the five basic sequences, T1, T2, FLAIR, DWI, and T2* in a very short time. The technical details of the sequence have already been published. The entire has eleven modules that can be used in various combinations to obtain a single-shot EPI-readout-based section for each weighting.[3] The utility of EPIMix was tested in two volunteers with promising results.[3] A previously published study found that, although EPIMix images had a lower quality, they had good diagnostic accuracy.[6] Another study found that EPIMix images had a lower quality with a higher incidence of susceptibility artifacts than conventional images but could supplement the conventional sequences in certain clinical scenarios.[7] The findings of the present concur with those of previous studies. The EPIMix-DWI was comparable to conventional sequences in terms of image quality and artifacts [Figure 2]. The rest of the sequences were sufficient for diagnostic use with a reduced overall quality. The most prominent reduction in the quality was seen in T2 and FLAIR images.
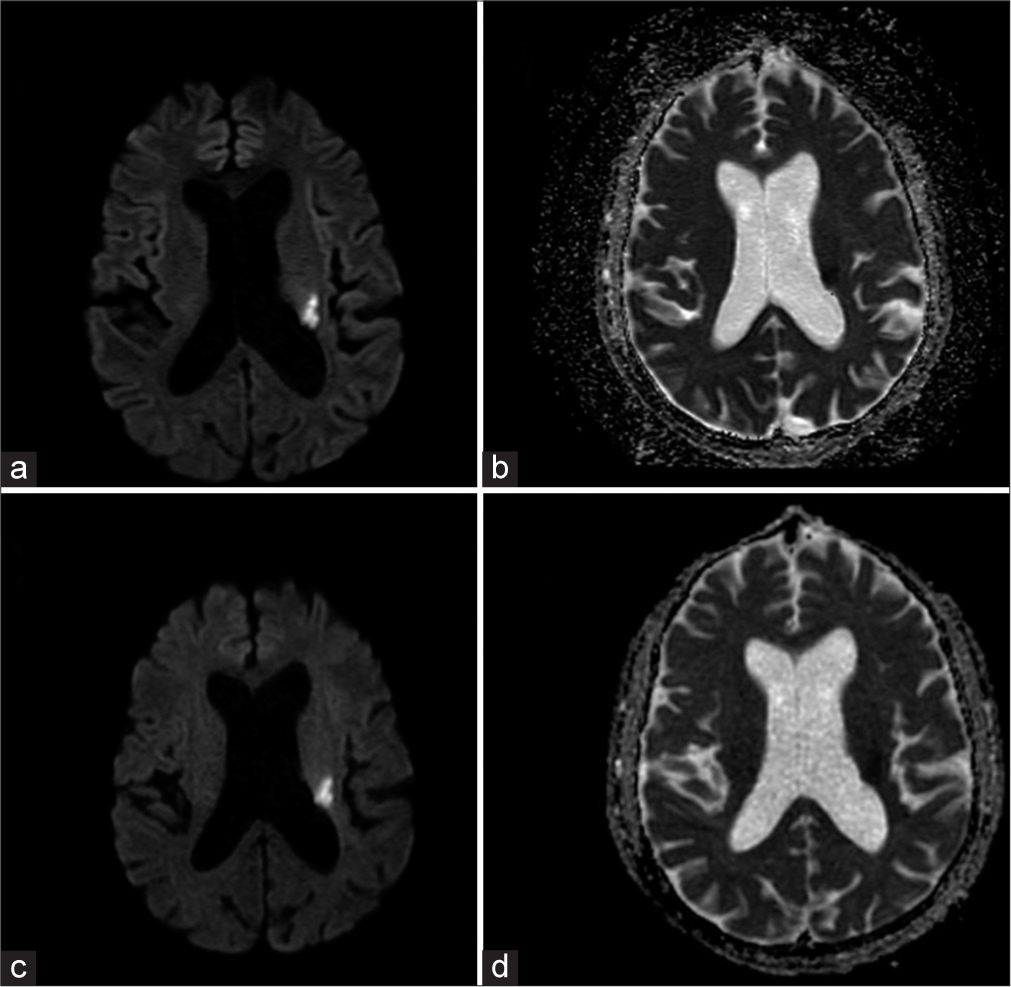
- A 68-year-old male patient with acute stroke presenting with sudden-onset weakness of the right upper and lower limbs. (a) Conventional-DWI and (b) ADC, (c) EPIMix-DWI, and (d) ADC axial MR images show that the image quality is comparable, and the acute infarct in the left corona radiata is well seen on the EPIMix images. DWI: Diffusion-weighted imaging, ADC: Apparent diffusion coefficient, EPIMix: Echo-planar imaging mix, MR: Magnetic resonance.
When we evaluated the diagnostic performance of EPIMix in various clinical scenarios, it was found that the sequence could be used to rule out the presence of any clinically relevant finding in many situations, as all patients with normal conventional MRI were reported as normal on EPIMix as well. Ischemic strokes were detected in all cases, likely on account of good-quality EPIMix-DWI images [Figure 2]. Intraparenchymal hemorrhages were also detected without difficulty [Figure 3]. The detection was poor for the smaller epileptogenic lesions and smaller vascular malformations, likely due to the lower resolution of EPIMix images. Among the intracranial tumors, identification of a possible residual or recurrent tumor adjacent to the operative bed proved to be difficult, probably due to the artifacts from the post-operative blood products. Tumors of the calvarium, skull base, and orbit both in pre-and post-operative scenarios were difficult to detect, again attributable to the increased susceptibility artifacts at air-bone-tissue interfaces [Figure 4]. Our results are concordant with the previous studies, where lesions were missed due to reduced resolution and increased artifacts.[6]

- A 46-year-old male patient presented with spontaneous intracranial hemorrhage. The patient was hypertensive and presented with headache and sudden onset left hemiparesis. (a) Conventional MR axial image with SWAN sequence and (b) Echo-planar imaging mix (EPIMix) axial image with T2* sequence. Although the EPIMix-T2* sequence is inferior in overall quality to SWAN, the acute right capsuloganglionic region hemorrhage is visualized. EPIMix: Echo-planar imaging mix, SWAN: Susceptibility-weighted angiography, MR: Magnetic resonance.
![A 14-year-old female patient with a right frontal calvarial lesion presenting with a swelling in the forehead on the right side. Conventional MR [(a) T1W, (b) T2W, (c) FLAIR, (d) DWI, and (e) SWAN] and EPIMix axial images [(f) T1W, (g) T2W, (h) FLAIR, (i) and (j) T2*] show the right frontal calvarial lesion (white arrow in a). Lesions of the skull base, calvarium, and orbit are more difficult to visualize on EPIMix images. T1W: T1 weighted, T2W: T2 weighted, FLAIR: Fluid-attenuated inversion recovery, DWI: Diffusion-weighted imaging, EPIMix: Echo-planar image mix, SWAN: Susceptibility-weighted angiography, MR: Magnetic resonance.](/content/150/2024/15/2/img/JNRP-15-341-g004.png)
- A 14-year-old female patient with a right frontal calvarial lesion presenting with a swelling in the forehead on the right side. Conventional MR [(a) T1W, (b) T2W, (c) FLAIR, (d) DWI, and (e) SWAN] and EPIMix axial images [(f) T1W, (g) T2W, (h) FLAIR, (i) and (j) T2*] show the right frontal calvarial lesion (white arrow in a). Lesions of the skull base, calvarium, and orbit are more difficult to visualize on EPIMix images. T1W: T1 weighted, T2W: T2 weighted, FLAIR: Fluid-attenuated inversion recovery, DWI: Diffusion-weighted imaging, EPIMix: Echo-planar image mix, SWAN: Susceptibility-weighted angiography, MR: Magnetic resonance.
We propose that EPIMix could either be performed in addition to or in place of conventional MRI sequences in certain clinical scenarios. In acute ischemic stroke, the conventional DWI image in our institute takes about 1 min and 8 s. Additional images such as FLAIR and T2* may also be obtained with no significant increase in the scan time if EPIMix is used, further helping streamline the treatment decisions. This could be extrapolated to acute hemorrhagic stroke, as substantiated by our study and previous studies, that have shown that GRE is comparable to CT in this setting.[17] Further, the presence of T1 and T2W images could help us determine the age of the hematoma. In other conditions, where the patient may be uncooperative, EPIMix could help us screen the extra-axial spaces and the neuroparenchyma for signs of pathology before performing the main MRI examination, which could be tailored to each patient. This may reduce the need for general anesthesia or sedation. One of the major problems of EPIMix could be in patients with postoperative or dental implants, due to the degree of susceptibility artifacts.
One of the limitations of our study was the subjective analysis of the image quality. Although the interobserver agreement was good in the present study, future studies may use objective measures to assess the signal-to-noise ratio and resolution. No cases with extra-axial hemorrhage or cranial trauma were evaluated. Magnetic resonance angiography, a vital part of acute stroke evaluation is not a part of EPIMix acquisition. A new sequence called NeuroMix has been introduced, which improves upon some of the shortcomings of EPIMix by adding FSE-based read-out for T2 and FLAIR sequences.[18] This may also be evaluated in the clinical setting by future studies.
CONCLUSION
The EPI-based sequence EPIMix has a significantly shorter acquisition time compared to the conventional MRI. The EPIMix-DWI was comparable to conventional sequences while the rest of the sequences showed a lower image quality, higher susceptibility artifacts, and reduced motion artifacts. In conditions like acute stroke, EPIMix could be used instead of the conventional MRI. In other clinical situations, EPIMix could supplement, rather than replace, the conventional imaging sequences.
Acknowledgments
This project was done under a research agreement with GE Healthcare. No financial support was obtained.
Author’s contributions
Dr. Viswanadh Kalaparti Sri Venkata Ganesh: Conceptualization, Methodology, Validation, Formal analysis, Investigation, Resources, Data curation. Writing– Original Draft, and Writing–Review and editing. Hari Kishore Kamepalli: Formal analysis, Resources. Dr. Dev Prakash Sharma: Formal analysis. Dr. Bejoy Thomas: Writing–Review and Editing. Dr. Chandrasekharan Kesavadas*: Conceptualization, Methodology, Resources, Writing–Review and Editing, Supervision, and Project administration.
Ethical approval
The research/study approved by the Institutional Review Board at Sree Chitra Tirunal Institute of Medical Sciences and Technology, number SCT/IEC/1806, dated January 2022.
Declaration of patient consent
Patient’s consent not required as patients identity is not disclosed or compromised.
Conflicts of interest
There are no conflicts of interest.
Use of artificial intelligence (AI)-assisted technology for manuscript preparation
The author(s) confirms that there was no use of artificial intelligence (AI)-assisted technology for assisting in the writing or editing of the manuscript and no images were manipulated using AI.
Financial support and sponsorship
Nil.
References
- Are movement artifacts in magnetic resonance imaging a real problem?-A narrative review. Front Neurol. 2017;8:232.
- [CrossRef] [PubMed] [Google Scholar]
- Motion artifacts in MRI: A complex problem with many partial solutions. J Magn Reson Imaging. 2015;42:887-901.
- [CrossRef] [PubMed] [Google Scholar]
- A 1-minute full brain MR exam using a multicontrast EPI sequence. Magn Reson Med. 2018;79:3045-54.
- [CrossRef] [PubMed] [Google Scholar]
- Ultrafast brain MRI: Clinical deployment and comparison to conventional brain MRI at 3T. J Neuroimaging. 2016;26:503-10.
- [CrossRef] [PubMed] [Google Scholar]
- Clinical feasibility of 1-min ultrafast brain MRI compared with routine brain MRI using synthetic MRI: A single center pilot study. J Neurol. 2019;266:431-9.
- [CrossRef] [PubMed] [Google Scholar]
- Diagnostic performance of a new multicontrast one-minute full brain exam (EPIMix) in neuroradiology: A prospective study. J Magn Reson Imaging. 2019;50:1824-33.
- [CrossRef] [PubMed] [Google Scholar]
- Clinical experience of 1-minute brain MRI using a multicontrast EPI sequence in a different scan environment. AJNR Am J Neuroradiol. 2020;41:424-9.
- [CrossRef] [PubMed] [Google Scholar]
- Sedation and general anaesthesia in children undergoing MRI and CT: Adverse events and outcomes. Br J Anaesth. 2000;84:743-8.
- [CrossRef] [PubMed] [Google Scholar]
- Cardiovascular MRI without sedation or general anesthesia using a feed-and-sleep technique in neonates and infants. Pediatr Radiol. 2012;42:183-7.
- [CrossRef] [PubMed] [Google Scholar]
- Parallel MR imaging: A user's guide. Radiographics. 2005;25:1279-97.
- [CrossRef] [PubMed] [Google Scholar]
- Gradient moment nulling for steady-state free precession MR imaging of cerebrospinal fluid. Med Phys. 1991;18:1038-44.
- [CrossRef] [PubMed] [Google Scholar]
- Motion correction with PROPELLER MRI: Application to head motion and free-breathing cardiac imaging. Magn Reson Med. 1999;42:963-9.
- [CrossRef] [Google Scholar]
- Principles and applications of echo-planar imaging: A review for the general radiologist. Radiographics. 2001;21:767-79.
- [CrossRef] [PubMed] [Google Scholar]
- Synthetic MRI in the detection of multiple sclerosis plaques. AJNR Am J Neuroradiol. 2017;38:257-63.
- [CrossRef] [PubMed] [Google Scholar]
- Comparison of MRI and CT for detection of acute intracerebral hemorrhage. JAMA. 2004;292:1823-30.
- [CrossRef] [PubMed] [Google Scholar]
- NeuroMix-A single-scan brain exam. Magn Reson Med. 2022;87:2178-93.
- [CrossRef] [PubMed] [Google Scholar]