Translate this page into:
Altered Expression of Heat Shock Protein-27 and Monocyte Chemoattractant Protein-1 after Acute Spinal Cord Injury: A Pilot Study
Akshay Anand, PhD Department of Neurology, Neuroscience Research Laboratory, Post Graduate Institute of Medical Education and Research Chandigarh India akshay1anand@redifmail.com
Vijay Goni, MS Department of Neurology, Neuroscience Research Laboratory, Post Graduate Institute of Medical Education and Research Chandigarh India vijaygoni@gmail.com
This article was originally published by Thieme Medical and Scientific Publishers and was migrated to Scientific Scholar after the change of Publisher.
Abstract
Abstract
Background Spinal cord injury (SCI) leads to serious complications involving primary trauma and progressive loss due to inflammation, local ischemia, or infection. Despite a worldwide annual incidence of 15 to 40 cases per million, methylprednisolone is the only treatment available to alleviate neurologic dysfunction; therefore, research is currently focused on identifying novel targets by biochemical and molecular studies.
Purpose Here, we investigated the expression of various molecular markers at the messenger ribonucleic acid (mRNA) and protein level at day 0 and day 30 post-SCI.
Methods Enzyme-linked immunosorbent assay (ELISA) was performed to determine the expression of CASPASE-3 and heat shock protein-27 (HSP-27) in serum samples. Real-time polymerase chain reaction (RT-PCR) was performed to determine the level of mRNA expression of vascular endothelial growth factor receptor-1 (VEGFR-1), VEGFR-2, HSP-27, monocyte chemoattractant protein-1 (MCP-1), and CASPASE-3.
Results HSP-27 expression at day 30, as compared with day 0, showed significant downregulation. In contrast, there was elevated expression of MCP-1. ELISA analysis showed no significant change in the expression of CASPASE-3 or HSP-27.
Conclusion There may be possible opposing role of HSP-27 and MCP-1 governing SCI. Their association can be studied by designing in vitro studies.
Keywords
angiogenesis
CASPASE-3
heat shock protein-27
monocyte chemoattractant protein-1
inflammation
molecular markers
spinal cord injury
vascular endothelial growth factor
vascular endothelial growth factor receptor-1
vascular endothelial growth factor receptor-2
Introduction
Injury to the spinal cord less than 3 weeks of age is considered acute spinal cord injury (SCI). SCI results in devastating complications, and the reversal of resulting deficits is a challenge for medical research. Despite extensive research to understand the pathophysiology of SCI, there exists no effective treatment that can reverse the deficits or interrupt the ongoing damage to the spinal cord following SCI.1Most SCIs are reported from high-velocity road traffic accidents, falls, crimes, and recreational activities with the incidence of injuries on the rise in geriatric populations.2Cervical spine is commonly affected as it is the most flexible region.3
A biphasic phenomenon best describes the pathophysiology of SCI. This includes a primary phase and secondary phase of injury.4The primary injury is caused by initial trauma, ischemia, demyelination, or infection. Further damage to the spinal cord continues in the secondary phase of injury characterized by tissue edema, electrolyte imbalance, cell death, free radical formation, excitotoxicity, chemotaxis, and immune cells infiltration.5Once initiated, all these mechanisms perpetuate a self-propagating cycle leading to deleterious consequences.
To stabilize the spinal column and prevent further damage, urgent intervention is required soon after surgery. Current treatments use a combination of medical, surgical, and rehabilitation therapy6although advantages from this combined intervention are not usually curative. Inflammation proceeds different phases. The phagocytic phase involves removal of debris from the site of injury followed by a proliferative phase characterized by revascularization aided by angiogenesis and extracellular matrix deposition, and finally, a modeling phase where wound retraction and tissue homeostasis are achieved.7 8 It has been suggested that secondary mechanisms may exacerbate complications, and therefore, controlling the secondary phase is also important for modifying the deficits.9 The identification of signal molecules is important to develop an understanding of the repair mechanisms. Current research is, therefore, focused on discovering newer molecular targets on which treatment modalities for acute SCI can be tested.
Many molecules are involved in injury mechanisms. Vascular endothelial growth factor (VEGF) has been studied in the pathogenesis of SCI and known to have dual neurotropic effects: by directly acting on the neurons to promote neurite extension and by activating glial cells that produce various growth factors promoting neuronal growth,10making it an attractive target for investigation in SCI.11 Similarly, the heat shock proteins (HSPs) are primarily released because of acute stress, and levels of expression of these highly conserved proteins are increased following SCI to preserve neuronal cells and repress chronic inflammation.12 13 14 Conversely, monocyte chemoattractant protein-1 (MCP-1) recruits cells to the site of injury that includes memory T cells, monocytes, and dendritic cells.15 In this respect, it is not clear whether recruited immune cells exacerbate tissue damage or promote repair16 but likely depend on the type of cells involved. A delicate balance between the two can be deciphered by sampling cerebrospinal fluid (CSF) at various time intervals.17 Furthermore, SCI and its long-term neurological deficits involve apoptosis of neurons and oligodendroglia in regions unaffected by the initial injury. This regulated apoptosis is executed through the caspase family of cysteine proteases.18
The aforementioned molecules are interrelated through various pathways and are involved in the pathogenesis of SCI. We, therefore, examined the role of these molecules in neuronal protection in acute SCI with the hope that this will result in the emergence of newer treatment targets for developing treatment modalities or predicting injury outcome.
Methods
Recruitment of Participants
All patients with acute traumatic SCI who presented to ATC emergency of the Post Graduate Institute of Medical Education and Research (PGIMER) trauma center in Chandigarh, India, between January 1, 2016, and February 26, 2017, were considered. Patients with sustained acute traumatic SCI with neurological deficits within a wide age group representing injury from all vertebrae levels were included in the study. Patients with any other comorbidities, injury to other organs, and without neurological deficits were excluded from the study. A total of 42 patients had met the inclusion criteria. All 42 patients were examined clinically and advised the requisite investigations with noncontrast computed tomography. The first samples for all 42 patients were taken in the emergency room and followed up after 30 days. Fourteen patients were lost in the follow-up and therefore excluded from the study. The remaining 28 patients were included in the study (Fig. 1).

-
Fig. 1 The work flow of the study conducted to estimate the expression levels of different genes. ELISA, enzyme-linked immunosorbent assay; HSP-27, heat shock protein-27; MCP-1, monocyte chemoattractant protein-1; VEGFR-1, vascular endothelial growth factor receptor-1.
Fig. 1 The work flow of the study conducted to estimate the expression levels of different genes. ELISA, enzyme-linked immunosorbent assay; HSP-27, heat shock protein-27; MCP-1, monocyte chemoattractant protein-1; VEGFR-1, vascular endothelial growth factor receptor-1.
Treatments Given
All patients underwent posterior decompression surgery with pedicle screw fixation for posterior spinal fusion. Surgery was done within 10 days from the date of injury. After surgery, all patients were additionally treated with aceclofenac 75 mg for 2 weeks, hydrocortisone 100 mg thrice daily for 5 days, and antibiotics (cephalosporin and amikacin) for 5 days.
Follow-Up Period
Each patient was followed up after 30 days, and blood samples were taken on the 1st day and 30th day. The first day was considered as the date of the presentation with injury in the hospital. At the 30th day, no neurological improvements were observed in any of the patients.
Ethical Committee Approval
The ethical approval for the recruitment of the participants and to conduct the study was taken from the Institutional Ethical Committee, PGIMER, Chandigarh, vide letter number NK/558/Res, dated February 4, 2014.
Sample Collection and Isolation of Peripheral Blood Monocytes, Plasma, and Serum
Five milliliters of blood was collected in a serum separator tube from SCI patients at day 1 (before intervention) and day 30. It was subjected to centrifugation for 15 minutes at 3000 rpm, and serum was collected. Blood was also collected in an ethylenediaminetetraacetate tube and kept at room temperature for ~2 to 3 hours to settle. The upper yellowish portion was collected and layered on an equal volume of Histopaque and centrifuged at 1800 rpm for 30 minutes. Finally, from the interphase of plasma and Histopaque, a buffy coat of peripheral blood mononuclear cells (PBMCs) was collected and stored in ribonucleic acid (RNA) later (Sigma Aldrich, United States), while plasma was collected in a separate vial and stored in-80°C ultrafreezer.
Enzyme-Linked Immunosorbent Assay
Enzyme-linked immunosorbent assay (ELISA) was performed to determine the protein expression in serum samples at both time points for CASPASE-3 and HSP-27. Analysis was made using commercially available ELISA kits (Genxbio). Briefly, serum samples were plated on a precoated antibody ELISA plates and incubated for 2 hours at 37°C. The washing with buffer was followed by secondary antibody incubation at room temperature for 1 hour. Absorbance was taken at 450 nm using ELISA reader as described by the manufacturer. Total protein concentration of samples was estimated using the Bradford method. A standard curve using bovine serum albumin was used as a protein standard, and ELISA concentrations were further normalized by their respective total protein concentrations.
RNA Isolation and cDNA Synthesis
PBMCs stored in RNA later were used for RNA isolation. Cells were washed using 1X PBS to remove RNA later, and then RNA isolation was performed using a commercially available kit (Qiagen, United States). RNA was used as a template to synthesize complementary deoxyribonucleic acid (cDNA) as per kit protocol (Thermo Scientific, United States). The expression of different genes was determined by subjecting cDNA to real-time polymerase chain reaction (RT-PCR) analysis (Applied Biosystems) using specific primers (Sigma, United States and Eurofins, Genomics) (Fig. 1).
Reverse Transcriptase Polymerase Chain Reaction
Marker gene expression was analyzed by RT-PCR. As angiogenic, inflammatory, and stress-related markers may change following SCI, the mRNA expression of VEGFR-1, VEGFR-2, HSP-27, and MCP-1 was normalized to B-actin housekeeping gene and subsequently quantified. The quantitative PCR data were analyzed using the method of Livak and Schmittgen19. The primer annealing temperature was optimized using gradient PCR validated by agarose gel electrophoresis. The samples were subjected to PCR analysis using specific primers. The relative fold change was determined for each sample. The primer details are shown in Table 1.
Gene name |
Forward primer sequence |
Reverse primer sequence |
Annealing temperature |
---|---|---|---|
Abbreviations: HSP-27, heat shock protein-27; MCP-1, monocyte chemoattractant protein-1; VEGFR-1, vascular endothelial growth factor receptor-1. |
|||
VEGFR-1 |
GCTGTGCGCGCTGCTT |
AACTCAGTTCAGGACCTTTTAATTTTGA |
63°C |
VEGFR-2 |
TGATACTGGAGCCTACAAGTGCTT |
CCTGTAATCTTGAACGTAGACATAAATGA |
58.9°C |
HSP-27 |
CGTGGTGGAGATCACTGGCAAGC |
CGGGCCTCGAAAGTGACCGG |
63°C |
MCP-1 |
5′-AGCAGCAAGTGTCCCAAAGA-3′ |
5′-TTGGGTTTGCTTGTCCAGGT-3′ |
64.2°C |
Statistical analysis
All the results were expressed as mean ± standard error of mean. The data were statistically analyzed using SPSS version 16.0. Data normality was analyzed using 1-KS sampling. The statistical significance of data was computed using the Mann-Whitney U test, and p < 0.05 was considered as statistically significant.
Results
Our study included 17 male patients (60.7%) and 11 female patients (39.3%) (Fig. 2A), suggesting higher incidence of SCI in male patients in accordance with the present trend.20 Minimum age of the patient was 17 years and the maximum was 65 years, with the mean age being 41.07 ± 13.711 years (Fig. 2B). Of 28 patients, 14 patients sustained injury to thoracic spine, 10 patients to lumbar spine, and 4 patients to cervical spine (Fig. 2C).

-
Fig. 2 (A) Pie chart showing gender distribution in the study. (B) Histogram showing age distribution. (C) Bar chart showing percentage-wise distribution of levels of vertebral injury.
Fig. 2 (A) Pie chart showing gender distribution in the study. (B) Histogram showing age distribution. (C) Bar chart showing percentage-wise distribution of levels of vertebral injury.
Protein Estimation
ELISA was performed to estimate change in protein levels of HSP-27 and CASPASE-3 after 1 month of follow-up. Protein estimation using ELISA showed no significant difference in the level of HSP-27 (p = 0.423) and CASPASE-3 (p = 0.979) between day 1 and day 30 (Fig. 3). We further analyzed the data and found that there was no significant change in both CASPASE-3 and HSP-27 in relation to age, gender, severity, and level of vertebrae involved (data not shown).

-
Fig. 3 Comparing (A) CASPASE-3 and (B) heat shock protein-27 expression in serum at day 0 and day 30 post-spinal cord injury. HSP-27, heat shock protein-27.
Fig. 3 Comparing (A) CASPASE-3 and (B) heat shock protein-27 expression in serum at day 0 and day 30 post-spinal cord injury. HSP-27, heat shock protein-27.
Gene Expression
VEGFR-2 expression was found to be elevated after 30 days of post-trauma to spinal cord; however, the increase was not statistically significant (p = 0.867). Similarly, expression of VEGFR-1 showed no significant change in the follow-up group (Figs. 4A, B). The relative expression of HSP-27 on day 1 was compared with day 30 (Fig. 5), and we found a significant decrease in the expression of HSP-27 (p = 0.001) at 30th day posttrauma. Expression of MCP-1 showed a significant elevation at 30th day posttrauma (Fig. 6).

-
Fig. 4 Fold change in gene expression levels of vascular endothelial growth factor receptor-1 (A) and vascular endothelial growth factor receptor-2 (B) at day 0 and day 30 post-spinal cord injury.
Fig. 4 Fold change in gene expression levels of vascular endothelial growth factor receptor-1 (A) and vascular endothelial growth factor receptor-2 (B) at day 0 and day 30 post-spinal cord injury.

-
Fig. 5 Fold change in gene expression levels of heat shock protein-27 at day 0 and day 30 post-spinal cord injury. *p > 0.05.
Fig. 5 Fold change in gene expression levels of heat shock protein-27 at day 0 and day 30 post-spinal cord injury. *p > 0.05.
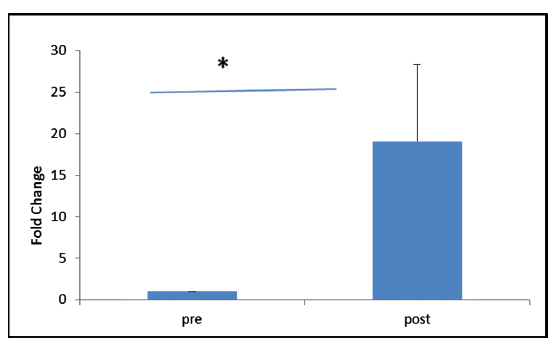
-
Fig. 6 Fold change in gene expression levels of monocyte chemoattractant protein-1 at day 0 and day 30 post-spinal cord injury. *p > 0.05
Fig. 6 Fold change in gene expression levels of monocyte chemoattractant protein-1 at day 0 and day 30 post-spinal cord injury. *p > 0.05
Discussion
SCI is a devastating condition with serious consequences. Proinflammatory and anti-inflammatory mechanisms participating in the secondary phase play a decisive role in the outcome.21 Different biomarker genes are expressed in a complex manner during secondary phase, but only a few of them are analyzed. The protective mechanisms include upregulation of regeneration-associated genes and neurotrophic factors.22 The present study was performed to probe any possible pattern in the expression of various angiogenetic biomarkers such as VEGFR-1, VEGFR-2, HSP-27, MCP-1, and CASPASE-3 in traumatic SCI so that the understanding of aforementioned secondary mechanisms could serve as a basis for devising new strategies for pharmacological interventions. Because of limited funds, ELISA could be performed only for CASPASE-3 and HSP-27.
VEGF promotes cell survival by reducing apoptosis and repairing blood vessel damage.23 Quantification of CASPASE-3 protein by ELISA post-SCI did not show any significant change in the posttraumatic period, which may suggest that CASPASE-3 is not altered by VEGFR, suggesting its redundant role in angiogenesis and neurogenesis post-SCI. These studies are in contrast to those performed by Voss et al,24 where VEGF has shown to have a nonredundant role. As discussed earlier, VEGF helps in restoration of blood vessels' damage by promoting angiogenesis and thereby inhibits the apoptotic machinery. VEGF exerts its angiogenic effects through its two major tyrosine kinases receptors, VEGFR-1 and VEGFR-2. Whereas VEGFR-1 recruits hematopoietic precursor cells and helps in the migration of monocytes and macrophages, VEGFR-2 plays a major role in the regulation of vascular endothelial cells.25 Quam et al26 reported the stimulation of VEGFR following ischemia resulting in the formation of newer vasculature and it has positive effects in promoting neurogenesis. Following neurological stress or trauma or any kind of injury, VEGFR is shown to be released by microglial, astrocytes, and monocytes as a compensatory response for inflammatory reactions and to protect neurons. Our study analyzed the PBMCs for any possible change in the expressions of both VEGFR-1 and VEGFR-2. As reported, the increase in expressions of both VEGFR-1 and VEGF-2, even though not significant, may exert neuronal protection and regeneration by promoting effects through angiogenesis. Interestingly, their increase can be antagonized by the inflammatory mechanisms following posttrauma, thus indicating the fine molecular balance that directs the cellular homeostasis. Insignificant change in the VEGF-1 and -2 can be due to delayed recovery, which can be investigated further with a long-term follow-up with sampling at intermittent intervals. Furthermore, antagonistic mechanisms to neuronal recovery like deposition of chondroitin sulfate at neuronal ends that occur in secondary phase after spine trauma can be contributory.27 A study done in rats found that the expression of VEGFR mRNA and protein levels get upregulated immediately following SCI; however, the levels get normalized after 14 days of SCI.28 Another study reported a significant decrease in VEGF levels after 1 day of surgery and it was maintained after 1 month post-SCI.29 HSP-27, being a stress protein and released from living cells on exposure to stress that occurs usually in chronic diseases, trauma, and infections, has been reported to be elevated after SCI and suggesting that it plays a key role in modulating secondary phase of spine injury by acting as a molecular chaperone and repairing the partially damaged neurons.14 Consistent with this study, a pre- and posttreatment study on rats with peroxisome proliferator-activated receptor inhibitors showed the protective neurological response that is due to elevation of HSP.30 31 There are additional studies with HSP-27 that shows its angiogenic and neurogenic potential. A few studies have also shown that the elevation of this protein following any kind of stress or tissue injury is associated with angiogenesis.32 In contrast to this, mutations in HSP 27 result in decreased expression of HSP and binding of heat shock factor to heat shock element resulting in impaired neuroprotection.33 34 We did not analyze any single nucleotide polymorphisms (SNPs) in HSP-27. In this context, a significant decrease in the levels of HSP-27 in the posttraumatic period indicates a subdued compensatory response. The cause of delayed activation could not be analyzed although HSP-27 and CASPASE-3 expression are often with apoptotic and angiogenic activity.
Similarly, the expression of MCP-1 in the acute phase of posttraumatic SCI was elevated within 30 days from the time of injury. This can be an intrinsic response to the inflammation caused after secondary SCI since MCP1 is released by monocytes, macrophages and dendritic cells in response to inflammatory reaction. A study in a rat model reported increased expression of MCP-1 in secondary SCI, due to inflammatory cytokines—interleukin-1β and tumor necrosis factor α. The same study reported that after anti-MCP-1 gene therapy, levels of MCP-1 expression, monocytes, and macrophage infiltration were reduced, further supporting inflammatory role of MCP-1. The reduction of MCP-1 expression is, therefore, protective during secondary SCI as it inhibits apoptotic process and reduces neuronal and astrocyte injury.35
It has been reported that the angiogenic role of MCP-1 through the p44/42 MAPK (Erk1/2) pathway upregulates hypoxia-inducible factor (e.g., VEGF) in the aortic endothelial cells.36 Upregulating the levels of VEGF-A also results in angiogenesis, pointing out a possible compensatory role by VEGF in SCI.37 It is possible that after SCI, there is activation of chemotactic activity resulting in an increase in the number of various chemotactic factors such as MCP-1, which further activates VEGFR (Fig. 7). We postulate that VEGFR-1 and VEGFR-2 and MCP-1 are important biomarkers released after SCI trauma and this is supported by other studies.31 38 They may exert their angiogenic influence to protect neurons from senescence and activate neuronal regeneration; however, their interaction with HSP-27 at other time points remains to be determined.

-
Fig. 7 Plausible mechanism of interaction of various biomarker genes in the secondary phase of spinal cord injury. HIF, hypoxia inducible factor; MCP, monocyte chemoattractant protein; VEGFR-1, vascular endothelial growth factor receptor-1.
Fig. 7 Plausible mechanism of interaction of various biomarker genes in the secondary phase of spinal cord injury. HIF, hypoxia inducible factor; MCP, monocyte chemoattractant protein; VEGFR-1, vascular endothelial growth factor receptor-1.
Limitations
The study consisted of a small sample size, and study participants were not studied with long-term follow-up. This study did not consider patients with any other comorbid conditions. The study was only limited to the group of patients who had undergone decompression surgery.
Conclusion
The decreased mRNA HSP-27 expression may indicate a subdued compensatory response or delayed activation, which needs further investigation at other time points. On the other hand, increased MCP-1 expression can constitute an intrinsic response to the inflammation caused after secondary SCI, suggesting a possible inverse association of HSP-27 and MCP-1 with SCI. These may be investigated as potential biomarkers in larger studies, where CSF samples can also be analyzed.
Conflict of Interest
None declared.
Funging None.
References
- Epidemiology, demographics, and pathophysiology of acute spinal cord injury. Spine. 2001;26(24):S2-S12. (suppl)
- [Google Scholar]
- A Clinical Practice Guideline for the Management of Acute Spinal Cord Injury: Introduction, Rationale, and Scope. Los Angeles, CA: SAGE Publications; 2017.
- [Google Scholar]
- Epidemiology of traumatic spinal cord injury: trends and future implications. Spinal cord. 2012;50(5):365.
- [Google Scholar]
- Translating mechanisms of neuroprotection, regeneration, and repair to treatment of spinal cord injury. Prog Brain Res. 2015;218:15-54.
- [Google Scholar]
- Emerging therapies for acute traumatic spinal cord injury. CMAJ. 2013;185(6):485-492.
- [Google Scholar]
- Degenerative and regenerative mechanisms governing spinal cord injury. Neurobiol Dis. 2004;15(3):415-436.
- [Google Scholar]
- Progressive inflammation-mediated neurodegeneration after traumatic brain or spinal cord injury. Br J Pharmacol. 2016;173(4):681-691.
- [Google Scholar]
- Ex vivo VEGF delivery by neural stem cells enhances proliferation of glial progenitors, angiogenesis, and tissue sparing after spinal cord injury. PLoS One. 2009;4(3):e4987.
- [Google Scholar]
- Recent molecular discoveries in angiogenesis and antiangiogenic therapies in cancer. J Clin Invest. 2013;123(8):3190-3200.
- [Google Scholar]
- The role of heat shock proteins Hsp70 and Hsp27 in cellular protection of the central nervous system. Int J Hyperthermia. 2005;21(5):379-392.
- [Google Scholar]
- Heat shock paradox and a new role of heat shock proteins and their receptors as anti-inflammation targets. Inflamm Allergy Drug Targets. 2007;6(2):91-100.
- [Google Scholar]
- The role of heat shock proteins in spinal cord injury. Neurosurg Focus. 2008;25(5):E4.
- [Google Scholar]
- Monocyte chemoattractant protein 1 acts as a T-lymphocyte chemoattractant. Proc Natl Acad Sci U S A. 1994;91(9):3652-3656.
- [Google Scholar]
- Repertoire of microglial and macrophage responses after spinal cord injury. Nat Rev Neurosci. 2011;12(7):388-399.
- [Google Scholar]
- Astrocytes initiate inflammation in the injured mouse spinal cord by promoting the entry of neutrophils and inflammatory monocytes in an IL-1 receptor/MyD88-dependent fashion. Brain Behav Immun. 2010;24(4):540-553.
- [Google Scholar]
- Activation of the caspase-3 apoptotic cascade in traumatic spinal cord injury. Nat Med. 1999;5(8):943-946.
- [Google Scholar]
- Analysis of relative gene expression data using real-time quantitative PCR and the 2- ΔΔCT method. Methods. 2001;25(4):402-408.
- [Google Scholar]
- Epidemiology of traumatic spinal cord injury: trends and future implications. Spinal Cord. 2012;50(5):365-372.
- [Google Scholar]
- Acute spinal cord injury, part I: pathophysiologic mechanisms. Clin Neuropharmacol. 2001;24(5):254-264.
- [Google Scholar]
- Degenerative and spontaneous regenerative processes after spinal cord injury. J Neurotrauma. 2006;23:264-280. (3/4)
- [Google Scholar]
- Vascular endothelial growth factor improves functional outcome and decreases secondary degeneration in experimental spinal cord contusion injury. Neuroscience. 2003;120(4):951-960.
- [Google Scholar]
- Binding of caspase-3 prodomain to heat shock protein 27 regulates monocyte apoptosis by inhibiting caspase-3 proteolytic activation. J Biol Chem. 2007;282(34):25088-25099.
- [Google Scholar]
- VEGF receptor signalling—in control of vascular function. Nat Rev Mol Cell Biol. 2006;7(5):359-371.
- [Google Scholar]
- VEGF-initiated blood-retinal barrier breakdown in early diabetes. Invest Ophthalmol Vis Sci. 2001;42:2408-2413.
- [Google Scholar]
- Rothman-Simeone The Spine E-Book: Expert Consult. Vol 1. Philadelphia Elsevier Health Sciences; 2011.
- [Google Scholar]
- Vascular endothelial growth/permeability factor in spinal cord injury. J Neurosurg. 1999;90(02):220-223.
- [Google Scholar]
- Reduced vascular endothelial growth factor expression in contusive spinal cord injury. J Neurotrauma. 2009;26(7):995-1003.
- [Google Scholar]
- Bone-marrow-derived mesenchymal stem cells promote proliferation and neuronal differentiation of Niemann-Pick type C mouse neural stem cells by upregulation and secretion of CCL2. Hum Gene Ther. 2013;24(7):655-669.
- [Google Scholar]
- Thiazolidinedione class of peroxisome proliferator-activated receptor gamma agonists prevents neuronal damage, motor dysfunction, myelin loss, neuropathic pain, and inflammation after spinal cord injury in adult rats. J Pharmacol Exp Ther. 2007;320(3):1002-1012.
- [Google Scholar]
- Extracellular HSP27 mediates angiogenesis through toll-like receptor 3. FASEB J. 2013;27(10):4169-4183.
- [Google Scholar]
- Human myocardium releases heat shock protein 27 (HSP27) after global ischemia: the proinflammatory effect of extracellular HSP27 through toll-like receptor (TLR)-2 and TLR4. Mol Med. 2014;20:280-289.
- [Google Scholar]
- Genetic variant in the HSPB1 promoter region impairs the HSP27 stress response. Hum Mutat. 2007;28(8):830.
- [Google Scholar]
- Inhibition of monocyte chemoattractant peptide-1 decreases secondary spinal cord injury. Mol Med Rep. 2015;11(6):4262-4266.
- [Google Scholar]
- Monocyte chemoattractant protein-1-induced angiogenesis is mediated by vascular endothelial growth factor-A. Blood. 2005;105(4):1405-1407.
- [Google Scholar]
- Induction of VEGF and VEGF receptors in the spinal cord after mechanical spinal injury and prostaglandin administration European. J Neurosci. 2000;12(10):3675-3686.
- [Google Scholar]
- Expression of inflammatory cytokines following acute spinal cord injury in a rodent model. Journal Neuroscience Res. 2012;90(4):782-790.
- [Google Scholar]